Unveiling How Microbes Sense and Navigate Their World
In the microscopic world teeming beneath our notice, bacteria orchestrate complex social behaviors that are vital both for their survival and for human health and industry. Among the most fascinating phenomena in microbiology is the formation of biofilms—structured bacterial communities adhering to surfaces, enveloped in self-produced extracellular matrices. These biofilms confer resilience and adaptability, often […]

In the microscopic world teeming beneath our notice, bacteria orchestrate complex social behaviors that are vital both for their survival and for human health and industry. Among the most fascinating phenomena in microbiology is the formation of biofilms—structured bacterial communities adhering to surfaces, enveloped in self-produced extracellular matrices. These biofilms confer resilience and adaptability, often complicating medical treatments and industrial processes. Recent research, led by Dr. Pushkar Lele at Texas A&M University, is unveiling the sophisticated sensory systems bacteria employ to navigate their physical environment. His work delves deeply into bacterial mechanosensing, a cutting-edge frontier in understanding how bacteria detect and respond to mechanical stimuli at the nano-scale to initiate surface colonization and biofilm development.
Mechanosensing in bacteria refers to the ability of these unicellular organisms to translate mechanical inputs into biochemical signals that trigger changes in gene expression and behavior. Unlike animals, bacteria lack specialized sensory organs, yet they achieve remarkable environmental awareness. Dr. Lele’s research focuses on revealing the molecular basis of this sensory transduction, particularly the role of specialized protein complexes embedded within bacterial membranes. These mechanosensors monitor mechanical forces such as shear stress and surface contact, serving as gatekeepers to the bacteria’s decision-making processes about colonizing new niches or engaging in pathogenic interactions.
Central to Lele’s investigation is the flagellar stator, a protein complex integral to the bacterial flagellum—a slender, rotary appendage that propels bacterial motility. The stator not only provides torque to spin the flagellum but also acts as a mechanosensor, detecting alterations in mechanical load when the bacterium encounters surfaces. This dual functionality is critical for a bacterium to ascertain whether it is free-swimming or has made contact with a surface, effectively flipping a molecular switch that sets off a cascade of intracellular signaling. The downstream effects of this signaling cascade include activation of biofilm-associated genes, initiation of genetic competence, and modulation of virulence factors.
Exploring these processes demands instrumentation capable of resolving structures at the scale of nanometers. Bacterial cells measure roughly one micron in size, with mechanosensors like the flagellar stator being an order of magnitude smaller. The precision required to detect and manipulate these molecular-scale phenomena has been achieved through the acquisition of advanced microscopy tools funded by the National Institute of General Medical Sciences (NIGMS). This technological leap enables the Lele Lab to visualize bacterial appendages and monitor mechanosensor activity in real time, providing unprecedented insight into the mechanical cues that drive microbial community dynamics.
The implications of understanding bacterial mechanosensing extend far beyond the confines of laboratory curiosity. From a medical perspective, bacteria coexist with humans as both beneficial microbiota and as opportunistic pathogens. Surface colonization by bacteria is a preliminary step in infection establishment, biofilm formation on tissues, and chronic disease progression. By elucidating the mechanosensory mechanisms that underpin these initial interactions, researchers aim to open new avenues for therapeutic intervention. For example, disrupting mechanosensor function could prevent harmful biofilms on implants or mucosal surfaces, potentially reducing antibiotic reliance.
In parallel, the industrial sector grapples with the economic burdens of biofouling—unwanted bacterial colonization of pipelines, filters, and machinery. Biofilms in industrial systems cause clogging, corrosion, and loss of efficiency, costing billions annually. Traditional anti-fouling strategies often rely on chemical treatments with ecological drawbacks. Insights from mechanosensing research could inform the design of surfaces or molecules that interfere with bacterial tactile sensing, thereby hampering their ability to adhere and form persistent communities without the need for harsh chemicals.
Dr. Lele emphasizes that the current research is not directly focused on creating novel probiotics or anti-biofouling agents. Rather, it seeks to formulate foundational knowledge about how microscopic life senses and adapts to its physical close-up world. This knowledge acts as a scientific foundation, igniting innovations across microbiology, bioengineering, and medicine. By decoding the language of mechanical signal transduction in bacteria, scientists may indirectly stimulate breakthroughs that transform healthcare and industrial practices.
The Lele Lab’s ongoing work is funded in part by a prestigious R01 grant from the NIH’s NIGMS division, an award first received when Dr. Lele was an assistant professor and recently renewed to continue advancing research in this domain. This sustained support underscores the value of fundamental biological research, which often yields unpredicted yet profoundly impactful results. Prior findings from this funding pathway include the identification of novel bacterial stress response pathways and mechanisms by which pathogens evade innate immune defenses, both critical insights that have shaped the broader field of microbiology.
Understanding the molecular details of bacterial mechanotransduction involves dissecting complex signaling pathways that intersect with genetic regulation. Binding events at mechanosensors trigger conformational changes in protein structures that propagate through signaling networks, modulating the activity of transcription factors and effectors controlling biofilm formation and bacterial competence. These intracellular pathways are intricate and species-specific, entailing multi-disciplinary approaches that blend biochemistry, structural biology, and biophysics.
The analogy often used to describe this molecular choreography is that of a hidden key in a grand piano, whose depression orchestrates a sweeping symphony. In bacteria, however, the instruments and performers are minuscule, invisible to the naked eye, yet their performances can influence macroscopic outcomes, from gut health to environmental contaminations. The challenge faced by Dr. Lele and his team is to characterize these mechanosensors’ molecular structures and dynamics, unlocking how mechanical signals are transduced into genetic and biochemical responses.
Flagellar stators remain a primary focus due to their clearly defined mechanical and signaling roles. Unlike sensory systems in higher organisms, these bacterial proteins straddle the physical and chemical domains, directly sensing mechanical impediments and converting these cues into second messenger signals inside the cell. Decoding this conversion process has immense potential for synthetic biology, where engineered microbes could be programmed with tunable responses to mechanical environments—applications ranging from smart biosensors to targeted microbial therapies.
The broader scientific community respects the Lele Lab’s interdisciplinary approach, combining advanced surface microscopy techniques with genetic manipulation and computational modeling. This synergy enhances understanding of bacterial ecology and pathogenesis within mechanical microenvironments previously inaccessible to experimental interrogation. The knowledge gained feeds into bigger questions on how physical forces shape life at all scales, redefining concepts of microbial behavior and evolution.
In conclusion, the burgeoning field of bacterial mechanosensing illuminated by Dr. Pushkar Lele’s research represents a nexus of microbiology, engineering, and medicine. Its revelations promise new insights into microbial life’s interaction with the physical world, with repercussions that resonate from cellular signaling to public health and industry. By harnessing state-of-the-art microscopy and molecular biology, the Lele Lab forges ahead in decoding the language bacteria use to “feel” their environment—a language that, once understood, could inspire innovative strategies to manipulate microbial communities beneficially or prevent their deleterious impacts.
Subject of Research: Mechanosensing in Bacteria and Surface Colonization Mechanisms
Article Title: Unlocking the Molecular Language of Bacterial Touch: Mechanosensing and Biofilm Formation
News Publication Date: Not specified
Web References:
Lele Lab: https://pushkarlelelab.org/
Review in Biomolecules: https://www.mdpi.com/2218-273X/15/3/355
References: NIH/National Institutes of Health funding acknowledgment
Keywords: Discovery research; Environmental methods; Industrial research; Health care industry; Public health; Intracellular signal transduction; Molecular targets; Industrial science; Biomedical research funding; Environmental illness; Biofilm formation; Bacterial signaling; Chemical signals; Chemical engineering; Downstream targets; Gene targeting; Surface microscopy; Cell membranes; Biofilm infections
Tags: bacteria navigation and environmental awarenessbacterial sensory systemsbiofilm formation in bacteriacomplex social behaviors of bacteriaextracellular matrices in microbiologyimplications of bacterial biofilms in health and industrymechanical stimuli response in bacteriamechanotransduction in unicellular organismsmicrobial mechanosensingprotein complexes in bacterial membranesresilience in microbial communitiessurface colonization by bacteria
What's Your Reaction?
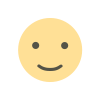
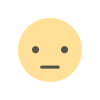
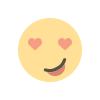
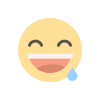
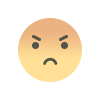
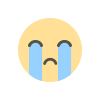
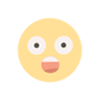