Transparent design advances high-performance flexible thermoelectric semiconductors
Scientists at Queensland University of Technology (QUT) have unveiled a groundbreaking development in the field of flexible semiconductors tailored for wearable electronics. Utilizing a cutting-edge approach known as vacancy engineering, these researchers have manipulated the atomic-scale structure of a novel AgCu(Te, Se, S) alloy, composed of silver, copper, tellurium, selenium, and sulfur, to create a […]

Scientists at Queensland University of Technology (QUT) have unveiled a groundbreaking development in the field of flexible semiconductors tailored for wearable electronics. Utilizing a cutting-edge approach known as vacancy engineering, these researchers have manipulated the atomic-scale structure of a novel AgCu(Te, Se, S) alloy, composed of silver, copper, tellurium, selenium, and sulfur, to create a flexible thermoelectric material. This material exhibits the remarkable ability to convert body heat into electricity efficiently, marking a significant advance toward powering wearable devices without reliance on batteries.
The innovation stems from the precise control and manipulation of atomic vacancies—small, intentional gaps where atoms are missing within the crystal lattice. Vacancy engineering allows scientists to dramatically alter and enhance a material’s electrical conductivity and mechanical flexibility by reorganizing these atomic voids. By tuning these microscopic absences, the QUT team has created an alloy that not only harnesses thermoelectric effects with higher efficiency but also exhibits superior ductility, expanding the potential for practical application in flexible and stretchable electronics.
Published in the esteemed journal Nature Communications, this research underscores the synergy between computational materials science and experimental methods. Guided by advanced computational design, the investigators developed a simple yet cost-effective melting synthesis technique, resulting in a flexible AgCu(Te, Se, S) semiconductor with unprecedented mechanical and thermoelectric performance. The dual enhancement of electrical generation from heat and mechanical resilience positions this semiconductor as a rare inorganic candidate with both outstanding conductivity and pliability.
Thermoelectric materials offer the unique advantage of converting thermal energy directly into electrical energy, which is particularly attractive for sustainable energy solutions. The human body itself presents a constant and accessible heat source, creating a natural temperature gradient with the environment that wearable thermoelectric devices can exploit. When a person exercises, the temperature differential further increases, generating more usable thermal energy that can be transformed into power for portable electronics, medical sensors, or communication devices.
Previously, the mainstream materials used for flexible thermoelectrics have been organic compounds or brittle inorganic thin films deposited on flexible substrates, each with inherent limitations. Organic semiconductors generally suffer from suboptimal electrical performance, while inorganic materials, though more efficient, tend to be rigid and prone to cracking under strain. The QUT team’s AgCu(Te, Se, S) semiconductor challenges this dichotomy by maintaining high thermoelectric efficiency alongside exceptional ductility, overcoming a long-standing barrier in the field.
A crucial breakthrough lies in the “vacancy engineering” technique, which the researchers applied to tailor the spatial distribution and concentration of atomic vacancies strategically. These vacancies influence the scattering of electrons and phonons within the crystal, optimizing the balance between electrical conductivity and thermal conductivity—a key metric in thermoelectric materials termed the figure of merit (ZT). By elevating this figure, the material achieves superior heat-to-electricity conversion rates without sacrificing flexibility.
The study highlights that the melting method employed for synthesis is both scalable and economically viable. This simplicity marks a departure from the often elaborate fabrication procedures common in semiconductor development, suggesting promising pathways for commercial manufacture. Moreover, the mechanical robustness endowed by vacancy-engineered microstructures supports repeated bending and stretching, crucial for wearable technology that must endure the dynamic motions of daily life.
This novel semiconductor has already been fashioned into micro-flexible devices designed for direct application on the human arm, effectively demonstrating the practical potential of the technology. These proof-of-concept prototypes illustrate how the material’s ability to conform to complex surfaces and maintain consistent thermoelectric output can revolutionize self-powered health monitoring systems and other wearable electronics, driving forward the vision of ubiquitous, battery-free sensing.
From a physics perspective, the underlying mechanisms of vacancy modulation in multicomponent chalcogenide alloys were largely unexplored until now. The QUT researchers’ elucidation of how vacancy patterns influence both electrical and mechanical properties fills a critical knowledge gap in the field of condensed matter physics and materials chemistry. By contributing foundational insights, this work opens avenues for rational design of other advanced flexible semiconductors with tailored functionalities.
QUT Professor Zhi-Gang Chen, a leading figure in this research, emphasized the rapidly growing demand for flexible thermoelectric devices in an era of wearable technology proliferation. “The key to advancing flexible thermoelectric technology,” he asserts, “lies in exploring a wide array of material systems and innovative fabrication techniques.” This comprehensive approach underpins the team’s success and sets a strategic direction for future advancements in zero-emission power generation.
This advancement aligns with QUT’s broader commitment to carbon neutrality and zero-emission energy solutions, with the project partly supported by the Australian Research Council’s Research Hub in Zero-emission Power Generation for Carbon Neutrality. Parallel efforts at QUT have also yielded ultra-thin, flexible films capable of powering next-generation wearable devices, emphasizing the institution’s position at the forefront of sustainable electronic materials research.
To further explore the full extent of this research and its implications, readers are encouraged to consult the original article titled Strategic vacancy engineering advances record-high ductile AgCu(Te, Se, S) thermoelectrics, available in Nature Communications via DOI: 10.1038/s41467-025-58104-x. The insights from this work promise to catalyze innovations in wearable electronics, flexible energy harvesters, and beyond, heralding a new era of environmentally friendly and mechanically adaptive thermoelectric technologies.
Subject of Research:
Not applicable
Article Title:
Strategic vacancy engineering advances record-high ductile AgCu(Te, Se, S) thermoelectrics
News Publication Date:
21-Mar-2025
Web References:
Nature Communications Article DOI
QUT School of Chemistry and Physics
QUT Centre for Materials Science
References:
Li, N.-H., Shi, X.-L., Liu, S., Cao, T.-Y., Zhang, M., Lyu, W.-Y., Liu, W.-D., Qi, D., & Chen, Z.-G. (2025). Strategic vacancy engineering advances record-high ductile AgCu(Te, Se, S) thermoelectrics. Nature Communications. https://doi.org/10.1038/s41467-025-58104-x
Image Credits:
QUT
Keywords
Thermoelectric materials, Environmental methods, Organic semiconductors, Thermoelectricity, Inorganic chemistry
Tags: advanced computational materials designAgCu(Teatomic-scale structure manipulationbody heat to electricity conversionductility in semiconductor materialsflexible thermoelectric semiconductorshigh-performance flexible materialsinnovative melting synthesis techniqueNature Communications publicationS) alloy developmentSethermoelectric efficiency enhancementvacancy engineering in materials sciencewearable electronics power solutions
What's Your Reaction?
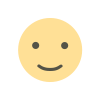
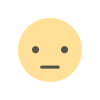
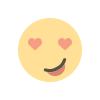
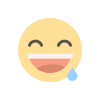
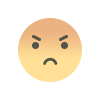
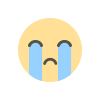
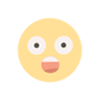