Scientists Discover Exotic Quantum Phase Once Considered Impossible
A team of researchers from Rice University has achieved a remarkable first: they directly observed a quantum phenomenon known as superradiant phase transition (SRPT), a theoretical concept that has been debated for over fifty years. This groundbreaking discovery may open new avenues for advancements in quantum computing, communication, and sensing technologies. By investigating the behaviors […]

A team of researchers from Rice University has achieved a remarkable first: they directly observed a quantum phenomenon known as superradiant phase transition (SRPT), a theoretical concept that has been debated for over fifty years. This groundbreaking discovery may open new avenues for advancements in quantum computing, communication, and sensing technologies. By investigating the behaviors of quantum particles in a unique crystalline structure, the researchers have provided evidence for a phenomenon that has long been thought impossible to observe in practical materials.
Superradiant phase transition arises when multiple groups of quantum particles synchronize their fluctuations to form a coherent state, effectively transitioning into a new state of matter. The researchers conducted their experiments using a crystal made from erbium, iron, and oxygen, which was cooled to an astonishing minus 457 degrees Fahrenheit while being subjected to an intense magnetic field of up to 7 tesla. This magnetic field is over 100,000 times stronger than Earth’s own magnetic force, demonstrating the extreme conditions under which such quantum phenomena might be explored.
Prominent in the research was Dasom Kim, a doctoral student at Rice, who led the investigative team. Kim explained that while the theory around SRPT suggested it could arise from interactions between quantum vacuum fluctuations and matter fluctuations, their work demonstrated that this transition could be initiated through the coupling of two distinct magnetic subsystems—the spins of iron and erbium ions within the crystal structure. This innovative approach effectively sidestepped the limitations imposed by the so-called “no-go theorem” that has long hampered experimental verification of such quantum theories.
The spins of electrons and particles play a crucial role in quantum mechanics, comparable to tiny arrows that exert a magnetic pull. When these spins align, they produce intricate magnetic patterns, much like waves rippling across a surface. This collective magnetic excitation is termed a magnon, representing a fundamental aspect of the interactions at play in quantum systems. Observing the formation of magnons under these conditions was essential to the team’s findings.
Using sophisticated spectroscopic techniques, researchers captured distinct signatures of the SRPT in their experiments. They noted that as they approached the superradiant phase, the energy signatures of specific spin modes displayed definitive changes; particularly, one mode’s energy dissipated while another’s exhibited a marked shift, or kink. These observations closely mirrored theoretical predictions of what should happen when entering the superradiant phase, instilling great confidence in the accuracy and integrity of the results.
The implications of this discovery extend far beyond academic interest. The ability to observe and understand collective quantum states during a superradiant phase transition holds transformative potential for the development of next-generation technologies. For instance, near this critical transition, the researchers discovered that the quantum system became capable of stabilizing quantum-squeezed states, which dramatically reduce quantum noise. This capability is crucial for enhancing the precision of quantum measurements, which is essential for advancing the functionalities of quantum sensors and computational devices.
The theoretical groundwork for this research was laid by Sohail Dasgupta, another graduate student at Rice, who worked alongside associate professor Kaden Hazzard, a co-author on the study. Dasgupta built on a mathematical model created by Motoaki Bamba, a professor at Yokohama National University. This collaboration highlights the fusion of experimental and theoretical physics, showcasing how theoretical predictions can be experimentally validated under the right conditions—a rare yet exhilarating experience for physicists.
Professor Hazzard emphasized the significance of this breakthrough, noting that it exemplifies the application of quantum optics concepts within solid materials. “This opens a new pathway for creating and manipulating phases of matter guided by the principles of cavity quantum electrodynamics,” he remarked. Such advancements could lead to the emergence of novel materials with tailored properties conducive to quantum applications.
The crystal employed in these studies is merely one instance of a broader category of materials. The findings pave the way for further investigations into quantum phenomena across various materials with comparable magnetic interactions. By establishing a form of SRPT driven through the coupling of internal matter fluctuations, the researchers have forged a fresh perspective in the field of quantum physics, providing a framework to understand and exploit inherent quantum interactions in materials.
Professor Junichiro Kono, who serves as the corresponding author of the study and is the Karl F. Hasselmann Professor in Engineering at Rice, conveyed the importance of their findings. “Demonstrating SRPT through the coupling of two internal fluctuations is a significant milestone in quantum physics. It establishes a new understanding of how matter can act in a quantum realm,” Kono stated. This work not only confirms a prediction that has lingered for decades but also highlights the possibilities for future exploration and advancements in quantum technologies.
As scientific inquiry continues to transcend established boundaries, the implications of the superradiant phase transition extend significantly into practical applications. The collective quantum states observed during such a transition could revolutionize the efficiency and capability of various quantum technologies, affecting areas from computing to sensor development. The enhanced measurement precision made possible by these states promises to elevate the fidelity of quantum experiments, laying a strong foundation for future innovation.
With the support of several prominent funding organizations—including the U.S. Army Research Office and the National Science Foundation—the researchers underline the collaborative effort involved in this cutting-edge work. This research was a cooperative endeavor among scientists from multiple institutions and disciplines, underscoring the nature of scientific advancement as a collective achievement rather than an individual pursuit.
This breakthrough in the observation of superradiant phase transitions not only enriches the tapestry of our understanding of quantum mechanics but also delineates clear pathways for the development of new technologies that harness quantum phenomena. The ability to fully comprehend and manipulate these quantum states marks a defining moment in physics, with potential ramifications that could alter the landscape of technological capabilities for years to come.
As we enter this exciting new chapter in the exploration of quantum behavior, the findings from Rice University stand as a testament to the perseverance and ingenuity of researchers dedicated to pushing the limits of human understanding. The journey has only just begun; as we strive to unlock more about the quantum world, the prospects for innovation are indeed limitless.
Subject of Research: Superradiant phase transition, quantum particles, and their implications in technology
Article Title: Observation of the magnonic Dicke superradiant phase transition
News Publication Date: April 11, 2025
Web References: Science Advances, Rice University News
References: Kim, D., et al. (2025). Observation of the magnonic Dicke superradiant phase transition. Science Advances. DOI: 10.1126/sciadv.adt1691
Image Credits: Photo by Jorge Vidal/Rice University
Keywords
Superradiance, quantum computing, quantum communication, quantum sensing, phase transition, quantum mechanics, optical properties, magnetic materials, experimental physics, collective excitation, spin dynamics, magnetic interactions.
Tags: advancements in quantum computing technologiesDasom Kim quantum researchextreme magnetic field experimentshistorical significance of quantum phase discoverynew states of matter in physicspractical observation of theoretical conceptsquantum communication breakthroughsquantum phenomena in crystalline structuresquantum sensing innovationsRice University research teamsuperradiant phase transitionsynchronization of quantum particles
What's Your Reaction?
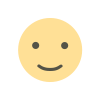
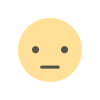
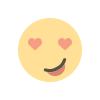
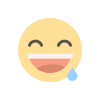
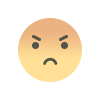
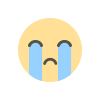
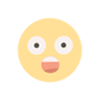