Enhanced RNA Editing Using Guide RNAs Mimicking ADAR
In the quest to harness the potential of gene editing for therapeutic applications, researchers have long recognized the necessity of approaches that balance precision, efficiency, and safety. One promising avenue that has emerged is RNA base editing via Adenosine deaminase acting on RNA (ADAR) enzymes. Unlike DNA genome editing, which involves permanent alterations to the […]

In the quest to harness the potential of gene editing for therapeutic applications, researchers have long recognized the necessity of approaches that balance precision, efficiency, and safety. One promising avenue that has emerged is RNA base editing via Adenosine deaminase acting on RNA (ADAR) enzymes. Unlike DNA genome editing, which involves permanent alterations to the genetic code, ADAR-mediated RNA editing offers a transient and reversible method to modulate gene expression at the RNA level. This distinction is crucial for clinical contexts requiring fine-tuned, nonpermanent interventions, reducing the risks associated with permanent genomic changes. Yet, despite its therapeutic allure, the efficiency of RNA editing tools, especially the design of guide RNAs (gRNAs) that recruit ADAR enzymes to specific sites, has posed challenges limiting their broad application.
Traditional RNA editing strategies employ guide RNAs featuring a fully complementary sequence to the target RNA but introduce a deliberate A–C mismatch at the target adenosine base. This mismatch is designed to prime the ADAR enzyme catalysis selectively at this site. However, accumulating biochemical evidence suggests that perfectly matched double-stranded RNA (dsRNA) structures do not represent the optimal substrate for ADAR enzymes; instead, certain secondary structures and sequence contexts in endogenous RNAs greatly influence editing efficiency. Addressing this limitation has been the focus of a recent landmark study by Sun, Cao, Song, and colleagues, who introduced an innovative approach called MIRROR—an acronym standing for mimicking inverted repeats to recruit ADARs using engineered oligoribonucleotides.
MIRROR represents a conceptual leap in gRNA design by integrating structural motifs derived from naturally occurring highly edited inverted Alu repeats within human tissues. Alu elements, short interspersed nuclear elements abundant in the human genome, frequently form double-stranded RNA structures through inverted repeats, which are substrates for extensive ADAR-mediated editing. By mimicking these inverted repeats, MIRROR-engineered gRNAs exploit the inherent affinity of ADAR enzymes for these specialized RNA structures, thereby enhancing targeted editing activity.
Importantly, the MIRROR strategy exhibits versatility across gRNA lengths and synthesis methods. The researchers demonstrated efficacy in both short, chemically synthesized gRNAs featuring nucleoside modifications intended to increase stability and long, biologically generated gRNAs derived from transcriptional processes. Such adaptability implies the approach can be fine-tuned for multiple delivery modalities, from synthetic oligonucleotides to viral vectors encoding longer RNA guides, addressing diverse therapeutic and experimental needs.
Quantitatively, the level of improvements conferred by MIRROR is striking. In human cell cultures spanning multiple cell types, editing efficiency was enhanced by up to 5.7-fold compared to conventional gRNA designs. This degree of enhancement was not confined to in vitro cell lines; primary hepatocytes from a mouse model of alpha-1 antitrypsin deficiency—a clinically relevant disease caused by misfolded proteins accumulating in the liver—also showed significant improvements in editing. The capacity to boost editing in primary patient-derived cells illustrates the translational potential of MIRROR for future therapeutic developments.
What distinguishes MIRROR beyond mere efficiency gains is its foundation in rational design principles grounded in natural RNA editing landscapes. The authors achieved this by extensive screening of endogenous RNA substrates that undergo high levels of ADAR editing in situ. Through this bioinformatic and structural analysis, they identified the critical architectural elements—such as bulges, mismatches, and stem-loop structures—that dictate ADAR binding and catalytic action. By incorporating these nuanced features into engineered gRNAs, MIRROR shifts the paradigm from empirical guesswork to informed, mechanistically guided RNA design.
The implications for programmable RNA editing are profound. Current state-of-the-art approaches, limited by static gRNA templates and suboptimal recruitment strategies, have struggled with low editing yields and off-target activity. The MIRROR framework provides a blueprint for next-generation editing tools that optimize on-target potency while maintaining specificity. This improvement is vital not only for experimental biology but also for therapeutic applications where safety and efficacy must be carefully balanced.
Furthermore, the study by Sun and colleagues advances the understanding of ADAR biology itself. By illustrating how specific structural motifs enhance enzymatic access and activity, MIRROR illuminates the broader mechanisms by which ADAR enzymes discriminate among RNA substrates in the cellular milieu. Such insights can fuel novel RNA editing platforms beyond ADAR, potentially catalyzing innovations across RNA biology and synthetic biology fields.
From a clinical development perspective, MIRROR’s improved guide RNA design could accelerate the translation of RNA base editing therapies for genetic disorders where aberrant RNA transcripts cause pathological phenotypes. Diseases such as alpha-1 antitrypsin deficiency, certain neurological conditions, and inherited metabolic disorders represent prime targets, particularly because RNA editing may correct pathogenic single nucleotide variants without altering the underlying DNA sequence permanently.
This strategy also addresses one of the critical safety concerns inherent in gene editing: irreversibility. By operating at the RNA level and relying on transient delivery of engineered gRNAs, MIRROR-based editing circumvents risks linked to permanent genome modifications, such as off-target DNA mutations, chromosomal rearrangements, or oncogenic transformations. This characteristic may foster greater regulatory acceptance and public trust for RNA editing therapeutics.
Technological integration with advances in delivery systems further enhances the appeal of MIRROR-based editing. Chemical modifications enabling enhanced nuclease resistance and targeted cellular uptake combined with viral or nonviral delivery vectors expand the clinical reach of these engineered gRNAs. As a result, in vivo editing in clinically relevant tissues becomes more feasible, potentially enabling treatment modalities administered via injection rather than invasive procedures.
The MIRROR approach also exemplifies the power of interdisciplinary collaboration, merging computational biology, structural RNA biochemistry, synthetic chemistry, and translational medicine. By reverse-engineering the principles underlying endogenous RNA editing processes, the team has illuminated a path toward more intelligent design of molecular tools with broad utility.
Looking forward, continued refinement of MIRROR and related RNA editing strategies will likely involve integrating CRISPR-based RNA targeting platforms, optimizing specificity profiles, and expanding the catalogue of editable nucleotide positions beyond the traditional adenosine deamination scope. Such progress promises a future where RNA editing serves as a programmable, tunable, and safe modality across a broad spectrum of diseases.
In sum, the advent of MIRROR marks a transformative step in RNA base editing technology. By co-opting evolutionary conserved RNA structural motifs to guide enzymatic activity, this method redefines how researchers can modulate RNA sequences efficiently and specifically. Its demonstrated improvements in editing efficiency across relevant experimental and disease models underscore its promise to significantly advance the field of therapeutic RNA engineering.
As the molecular biology community embraces MIRROR and its underlying principles, it is conceivable that RNA editing will soon rival or complement genome editing in clinical relevance. Safe, reversible, and highly efficient RNA modulation could open unprecedented opportunities for personalized medicine and the treatment of genetic diseases hitherto deemed intractable.
The year 2025 may well be remembered as a watershed moment when the synergy between natural RNA regulatory architectures and synthetic molecular engineering gave rise to a new era of precision medicine technology—one where ephemeral edits at the RNA level pave the way to durable health outcomes.
Subject of Research: RNA base editing; ADAR enzyme recruitment; guide RNA engineering; therapeutic RNA editing.
Article Title: Improved RNA base editing with guide RNAs mimicking highly edited endogenous ADAR substrates.
Article References:
Sun, Y., Cao, Y., Song, Y. et al. Improved RNA base editing with guide RNAs mimicking highly edited endogenous ADAR substrates. Nat Biotechnol (2025). https://doi.org/10.1038/s41587-025-02628-6
Image Credits: AI Generated
Tags: ADAR enzyme applicationsbiochemical mechanisms of RNA editingefficiency challenges in RNA editingendogenous RNA sequence contextsguide RNA design strategiesnonpermanent genetic interventionsprecision in RNA therapeutic applicationsreversible RNA editing techniquesRNA base editingsecondary structures in RNA editingtherapeutic gene editing approachestransient gene expression modulation
What's Your Reaction?
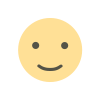
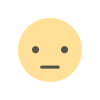
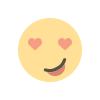
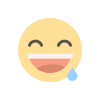
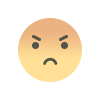
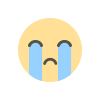
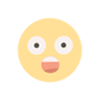