Light-Driven Phagobot Excels In Vitro and In Vivo
In a groundbreaking development that promises to redefine the frontiers of targeted therapy and biomedical interventions, researchers have introduced an innovative class of microrobots powered by light and inspired by the innate biological functions of macrophages. These pioneering phagocytic macrophage microrobots, affectionately dubbed “phagobots,” seamlessly blend the complexity of living cells with cutting-edge engineering to […]

In a groundbreaking development that promises to redefine the frontiers of targeted therapy and biomedical interventions, researchers have introduced an innovative class of microrobots powered by light and inspired by the innate biological functions of macrophages. These pioneering phagocytic macrophage microrobots, affectionately dubbed “phagobots,” seamlessly blend the complexity of living cells with cutting-edge engineering to achieve unprecedented control and efficacy in navigating complex biological landscapes. This fusion of biology and technology heralds a new era in minimally invasive medical treatments, where tiny, autonomous agents could seek out and neutralize disease with remarkable precision.
At the heart of this breakthrough lies a meticulous design strategy that leverages the unique properties of macrophages—immune cells notorious for their phagocytic capabilities, which enable them to engulf pathogens and cellular debris. By harnessing these cells’ innate functions, the research team has endowed phagobots with the extraordinary ability to not only traverse cellular environments autonomously but also to carry out targeted destruction of harmful materials. The integration of light-powered propulsion systems turns these biological machines into active agents capable of controlled movement without the need for external mechanical parts, reducing the risk of immune rejection or toxicity.
One of the most compelling aspects of the phagobot technology is its reliance on light as an energy source. Utilizing specific wavelengths, these microrobots are activated and guided, enabling on-demand control over their movement and function. This method sidesteps the limitations of chemical fuels, which often introduce complications in biological settings. The use of light provides a non-invasive, highly tunable stimulus that can penetrate deep enough into tissues to direct the microrobots while minimizing collateral damage to surrounding cells. This precision is vital in applications such as targeted drug delivery and clearing diseased or necrotic tissues.
The engineering of the phagobot involved delicate cellular manipulations to confer them with photoreactive capabilities. This was achieved by integrating nanoscale photosensitive materials within the membranes of macrophages, effectively turning these immune cells into microscopic robots responsive to external light cues. When illuminated, these materials induce localized biochemical reactions that generate propulsion forces, allowing the phagobots to swim, steer, and adjust their velocity within fluidic biological environments. This transformation from a passive phagocyte into an agile microrobot is a feat of multidisciplinary innovation combining cell biology, materials science, and photonics.
In vitro experiments with phagobots demonstrated remarkable dexterity in movement and the ability to selectively phagocytose target particles, including bacterial pathogens and synthetic debris mimicking diseased tissues. By illuminating the microrobots with controlled light patterns, the researchers successfully navigated them through microscopic mazes and fluid channels, mimicking the complex vasculature of human tissues. The phagobots exhibited targeted accumulation in predesignated areas, showcasing their potential for site-specific therapeutic interventions that minimize systemic side effects and enhance treatment efficacy.
The transition from in vitro to in vivo studies marked a critical milestone. Upon injection into animal models, phagobots retained their mobility and phagocytic function within living organisms, overcoming biological barriers that often hinder microrobot navigation. Their biocompatibility was a significant advantage, as these cellular machines are less likely to provoke adverse immune responses compared to synthetic counterparts. Fluorescence imaging and histological analyses confirmed the microrobots’ capacity to home in on sites of infection or inflammation, providing a promising platform for the development of targeted immunotherapies and pathogen clearance mechanisms.
Beyond infection control, the phagobot technology holds transformative potential for tackling cancerous tissues. Tumors often create immunosuppressive microenvironments, making it challenging for conventional therapies to be effective. Phagobots, armed with their ability to recognize and engulf abnormal cells, could be programmed to discriminate malignant from healthy cells, delivering cytotoxic payloads precisely where needed. Furthermore, their light-responsive propulsion ensures versatile maneuverability, allowing deep penetration into tumor masses that are typically difficult for drugs to reach, thereby overcoming one of oncology’s longstanding hurdles.
The modular nature of phagobot design also permits a high degree of customization. By varying the type of light-sensitive nanomaterials and adjusting illumination parameters, the researchers can fine-tune phagobot behavior—including speed, directionality, and phagocytic activity. This adaptability opens avenues for personalized medicine, tailoring microrobot functions to the specific needs of individual patients or disease states. The integration of biosensors within phagobots further enables real-time monitoring of their environment, providing critical feedback that can be used to optimize therapeutic outcomes dynamically.
Safety remains paramount in the advancement of any biomedical technology, and the phagobot development is no exception. Comprehensive toxicity assays demonstrated that the light intensities employed do not induce significant tissue damage or cellular stress, making the approach highly compatible with living systems. Moreover, since the phagobots are derived from native immune cells, they exhibit natural degradation pathways, mitigating concerns about accumulation or long-term persistence. The researchers emphasize that ongoing work aims to establish robust protocols for clearance and control post-treatment to ensure patient safety.
The integration of phagobot technology with existing medical equipment holds promise for clinical translation. For example, coupling these microrobots with endoscopic light delivery systems could allow physicians to precisely deploy and guide them during minimally invasive procedures. Additionally, the ability to remotely activate phagobots using external light sources means that patients could potentially receive treatments without extended hospital stays, heralding a new frontier in outpatient care and telemedicine. The versatility and user-friendly nature of the system bode well for future scalability and widespread adoption.
Challenges remain, of course, in scaling production and ensuring consistent functionality across batches of phagobots. The manufacturing process requires tight control over cellular modifications and material integration to preserve cell viability and responsiveness. The research team is exploring automated biofabrication techniques and advanced quality control measures to address these concerns. Moreover, regulatory pathways for cellular microrobots are still nascent, necessitating thorough documentation of safety and efficacy to obtain approvals. Nonetheless, the demonstrated success in preclinical models provides a strong foundation for optimistic progress.
The conceptual leap represented by the phagobot underscores a broader trend in nanomedicine: the integration of living cells as active components in therapeutic devices. Unlike traditional synthetic nanocarriers, these hybrid systems capitalize on biological intelligence and adaptability, offering dynamic responses to complex physiological cues. As the repertoire of biological microrobots expands, new horizons in disease diagnosis, targeted therapy, and regenerative medicine emerge, driven by the unique synergy of nature and technology.
Looking forward, the researchers envision expanding phagobot capabilities beyond phagocytosis to encompass drug nanocarrier functions, genetic modification delivery, and immune modulation. Incorporating artificial intelligence algorithms to autonomously navigate complex environments or respond to biochemical signals could further enhance their utility. As this field progresses, the ethical and societal implications will likewise need careful consideration, ensuring that the deployment of living microrobots aligns with safety standards and public trust.
In conclusion, the advent of light-powered phagocytic macrophage microrobots marks a seminal advancement in biomedical technology, combining cellular biology’s sophistication with cutting-edge engineering to create novel therapeutic agents. Their demonstrated effectiveness both in vitro and in vivo paves the way for revolutionary strategies in combating infections, cancer, and other diseases resistant to conventional approaches. As the research community builds upon these foundational achievements, the vision of intelligent, autonomous microrobots operating within the human body moves closer to reality, promising transformative impacts on healthcare and beyond.
—
Subject of Research: Development and application of light-powered phagocytic macrophage microrobots for targeted biomedical interventions.
Article Title: Light-powered phagocytic macrophage microrobot (phagobot): both in vitro and in vivo.
Article References:
Li, X., Zhong, S., Pan, T. et al. Light-powered phagocytic macrophage microrobot (phagobot): both in vitro and in vivo. Light Sci Appl 14, 202 (2025). https://doi.org/10.1038/s41377-025-01881-3
Image Credits: AI Generated
DOI: https://doi.org/10.1038/s41377-025-01881-3
Tags: autonomous cellular agentsbiological machines for disease treatmentbiomedical interventions advancementscontrolled movement in biomedicineengineered immune cell applicationslight-driven microrobotsmacrophage-inspired microrobotsminimally invasive medical treatmentsphagobot design and functionalityphagocytic macrophage technologyprecision medicine with microrobotstargeted therapy innovations
What's Your Reaction?
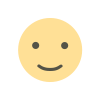
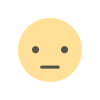
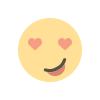
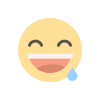
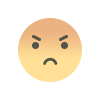
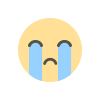
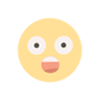