Transverse Mode Coupling in Few-Mode Fiber Lasers
In a groundbreaking development set to revolutionize fiber laser technology, a team of researchers led by B. Rao, J. Chen, and Z. Wang has unveiled novel insights into transverse mode coupling in monolithic few-mode fiber laser oscillators. Published in Light: Science & Applications, their comprehensive study sheds light on the complex interplay of modal dynamics […]

In a groundbreaking development set to revolutionize fiber laser technology, a team of researchers led by B. Rao, J. Chen, and Z. Wang has unveiled novel insights into transverse mode coupling in monolithic few-mode fiber laser oscillators. Published in Light: Science & Applications, their comprehensive study sheds light on the complex interplay of modal dynamics within these compact laser systems, promising advancements in both performance and application versatility. This research not only deepens our fundamental understanding of light-matter interactions in few-mode fibers but also paves the way for innovations in telecommunications, sensing, and high-power laser design.
Few-mode fiber lasers have attracted significant attention over recent years due to their potential to combine the high beam quality of single-mode lasers with the enhanced power handling capabilities of multimode systems. Unlike traditional single-mode fibers that support only one spatial mode, few-mode fibers allow a controlled number of transverse modes, offering a rich platform for complex mode interactions. However, this modal multiplicity inherently leads to challenges including mode competition, instability, and nonlinear coupling effects that can degrade laser performance. The current study focuses intensively on the transverse mode coupling phenomena within monolithic few-mode fiber laser oscillators—a critical aspect dictating the spatial coherence and stability of the emitted laser beam.
The term ‘monolithic’ refers to the integration of the laser cavity directly into a continuous fiber structure without discrete components such as gratings or mirrors fabricated separately. This integration simplifies device architecture, improves robustness, and enhances vibration resistance, but also complicates modal control since the laser modes evolve intrinsically along the fiber length. Rao and colleagues embarked on a rigorous theoretical and experimental investigation to map the underlying physics governing transverse mode coupling, primarily arising from subtle perturbations and nonlinear optical effects present in the fiber medium.
Central to their analysis is the coupling between the fundamental LP01 mode and higher-order modes such as LP11 and LP21, which coexist in the few-mode fibers used. The researchers employed advanced numerical simulations based on coupled-mode theory augmented by nonlinear Schrödinger equations to model the spatial and temporal evolution of the laser modes. These computational studies revealed intricate energy transfer dynamics, where modal gain competition, stimulated Raman scattering, and Kerr nonlinearities interplay to create complex oscillation patterns. Importantly, the monolithic configuration amplified some coupling channels while suppressing others, highlighting the delicate balance between structural design and nonlinear effect management.
Complementing the theoretical work, the team fabricated state-of-the-art few-mode fiber laser resonators engineered to exhibit precise core and cladding profiles optimizing modal discrimination. Using robust pump setups and sensitive modal-resolved detection techniques, they experimentally observed clear signatures of transverse mode coupling manifested as intensity fluctuations and spatial beam profile variations. These experimental validations provided crucial confirmation of the predicted coupling mechanisms and afforded feedback for iterative refinement of the fiber design parameters.
One of the most striking outcomes of the study is the identification of conditions under which controlled transverse mode coupling can be harnessed beneficially rather than suppressed. By fine-tuning the pump power, fiber geometry, and refractive index profile, it is possible to stabilize desired mode combinations, enhancing beam shaping flexibility and enabling dynamic mode control. This opens exciting prospects for custom-tailored fiber lasers capable of switching beam patterns on demand, which is invaluable for applications like optical trapping, material processing, and high-resolution microscopy.
Furthermore, the findings highlight the potential for scaling up output power without sacrificing beam quality by managing mode coupling proactively. Typically, high-power fiber lasers are plagued by mode instabilities resulting in beam degradation, but the new insights enable more effective design strategies that mitigate these instabilities. This advance could significantly impact industrial and scientific applications requiring reliable, high-brightness laser sources, such as precision cutting, additive manufacturing, and coherent lidar systems.
The introduction of monolithic architecture combined with deliberate modal engineering also reinforces the prospects for miniaturized, integrated photonic devices. Compact few-mode fiber lasers with robust mode control mechanisms are ideal candidates for on-chip integration in optoelectronic circuits, quantum communication networks, and advanced sensor arrays. Integration reduces system complexity and enhances portability, which is critical for next-generation technologies requiring distributed or field-deployable laser sources with stable performance.
Another key implication from Rao and the team’s work is the enhanced understanding of nonlinear optical phenomena in few-mode systems, particularly how Kerr nonlinearities modulate mode coupling dynamics under high-intensity operation. By dissecting these interactions, the research provides foundational knowledge applicable to managing supercontinuum generation, frequency combs, and soliton formation within multimode fibers. Such nonlinear processes are of great interest both fundamentally and technologically, underpinning various cutting-edge photonics developments.
Despite these advances, the authors acknowledge ongoing challenges related to environmental sensitivity and thermal effects that can provoke unwanted mode drift or coupling fluctuations. Addressing these issues will require further innovations in fiber material composition, thermal management, and active feedback control systems, signaling a rich direction for future investigations. Nonetheless, this research lays a robust groundwork upon which enhanced monolithic few-mode fiber lasers can be systematically designed and optimized.
In summary, this study represents a landmark achievement in the physics and engineering of few-mode fiber lasers. By unraveling the nuanced mechanisms of transverse mode coupling within monolithic oscillators, Rao and colleagues propel the field toward more powerful, stable, and versatile laser sources. Their work not only advances the state of knowledge but also aligns with practical objectives in diverse scientific and industrial domains, promising significant technological impact.
Looking ahead, continued exploration of mode coupling phenomena in more complex fiber architectures, including multicore and hybrid fibers, may yield even richer modal landscapes and novel functionalities. Integrating machine learning and adaptive optics techniques could further enhance dynamic mode control and stability, making these lasers smarter and more responsive to user requirements.
As photonics continues to drive innovation in communication, sensing, and manufacturing, the ability to engineer mode interactions at the fiber laser level becomes increasingly critical. The pioneering insights provided in this comprehensive study offer a compelling roadmap for how monolithic few-mode fiber lasers can be harnessed to meet these evolving demands, ultimately contributing to the advancement of high-performance laser technologies worldwide.
Subject of Research: Transverse mode coupling dynamics in monolithic few-mode fiber laser oscillators.
Article Title: Transverse mode coupling in monolithic few-mode fiber laser oscillators.
Article References:
Rao, B., Chen, J., Wang, Z. et al. Transverse mode coupling in monolithic few-mode fiber laser oscillators. Light Sci Appl 14, 187 (2025). https://doi.org/10.1038/s41377-025-01862-6
Image Credits: AI Generated
DOI: https://doi.org/10.1038/s41377-025-01862-6
Tags: beam quality in laser systemsfew-mode fiber lasershigh-power laser designLight-matter interactionsmodal dynamics in fiber lasersmode competition challengesmonolithic fiber laser oscillatorsmultimode fiber capabilitiesnonlinear coupling effects in laserssensing applications in fiber opticstelecommunications advancementstransverse mode coupling
What's Your Reaction?
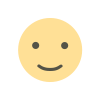
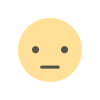
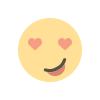
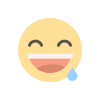
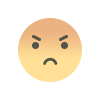
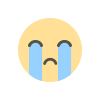
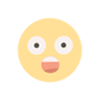