TIFRH Researchers Discover How Glasses Can Self-Regulate Their Brittleness
Materials that adjust their mechanical properties autonomously have long intrigued scientists aiming to develop smarter, more responsive systems. Now, researchers at the Tata Institute of Fundamental Research (TIFR) in Hyderabad have uncovered a compelling mechanism by which such adaptive behavior emerges naturally in active glasses—disordered materials composed of self-driven components. Their computational simulations open new […]

Materials that adjust their mechanical properties autonomously have long intrigued scientists aiming to develop smarter, more responsive systems. Now, researchers at the Tata Institute of Fundamental Research (TIFR) in Hyderabad have uncovered a compelling mechanism by which such adaptive behavior emerges naturally in active glasses—disordered materials composed of self-driven components. Their computational simulations open new perspectives on how biological tissues might regulate their mechanical state and inspire the design of innovative synthetic materials with tunable rigidity and ductility.
Glasses, unlike crystalline solids with orderly atomic layouts, are amorphous and lack periodic structure. Atoms or particles in glasses are arranged without long-range order, which imbues these materials with unique mechanical characteristics. When the constituent particles possess internal energy sources enabling autonomous movement, the material qualifies as an active glass. Such systems are not abstract theoretical constructs: they aptly model collections of living cells in tissues or bacterial colonies where chemical energy powers persistent internal activity.
A crucial property of glasses, active or passive, is their strong dependence on thermal history or preparation protocols. Glass formation involves rapidly cooling a liquid to bypass crystallization, leading to a “supercooled” liquid phase. Upon further cooling past a characteristic glass transition temperature, the system’s dynamics become enormously sluggish, crossing into a kinetically arrested state. Despite this dramatic slowdown in motion, the structural arrangement remains largely unchanged, embedding the system in a disordered but effectively frozen configuration.
This dependence on preparation rates imparts glasses with history-dependent mechanical responses. Slow cooling typically yields well-annealed glasses that are brittle, fracturing suddenly under stress. Conversely, rapid quenching traps the system in higher energy states, giving rise to ductile glasses capable of plastic deformation and localized necking before failure. Visualizing this through the energy landscape metaphor, glasses reside in local minima separated by energy barriers—the deeper the minimum, the more stable and brittle the glass becomes.
In their landmark simulations, Sharma and Karmakar have shown that inducing activity—self-motility—in components of a poorly annealed glass effectively acts as a dynamic annealing process. This local rearrangement driven by internal forces helps the system escape shallow traps, gradually lowering its overall potential energy. Remarkably, this process transitions the material from a ductile regime into a brittle one, highlighting how internal activity can steer the energy landscape navigation more efficiently than passive thermal equilibration.
This insight has profound implications, not only for materials science but also for biology. The increased aging and hardening observed in active glasses mirror the mechanical evolution of biological tissues during maturation and wound healing. The fact that living tissues inherently consist of active cells suggests that biological systems might exploit such activity-induced annealing to regulate their mechanical properties dynamically over time. This realization could inspire engineered metamaterials that integrate internally active constituents to achieve tunable durability and stiffness.
Beyond mechanical annealing, the study draws parallels between active glasses and their passive counterparts subjected to oscillatory shear deformations. Oscillatory shear involves cyclically straining a material back and forth, a process well-studied in amorphous solids to probe their yielding and memory effects. The researchers discovered that the amplitude and frequency of applied oscillations in passive glasses map quantitatively onto the strength and persistence of active forces in active glasses, effectively unifying these seemingly disparate processes.
Repeated cyclic shear is known to imprint “memories” in passive glasses, where the system’s mechanical response encodes information about the shear amplitude. Astonishingly, analogous memory effects were detected in active glasses using adapted protocols, suggesting that internal activity endows these systems with a form of mechanical learning. Given that cellular activity is intimately linked to metabolism, these findings hint at a novel connection between energy consumption, mechanical history, and adaptive behavior in biological tissues.
Further parallels include the transition from a jammed or stuck state to a fluidized state under strong driving forces. In both oscillatory shear and active glasses, increasing the driving amplitude or active force leads to fluidization, enabling large-scale particle rearrangements. This fluidization phenomenon is vital to understand biological processes such as morphogenesis and wound healing, where controlled fluid-like cell migration underpins tissue remodeling and repair.
What makes this study particularly transformative is the effective mapping it establishes between active glasses and oscillatory-driven amorphous solids. Researchers can now import the extensive theoretical and experimental toolkit developed for passive glasses under shear to unravel the complex behavior of active biological matter. This cross-pollination promises breakthroughs in understanding collective cell migration, tissue mechanics, and the design of bioinspired materials with programmable responses.
Nevertheless, it is important to note that while activity-induced annealing significantly enhances equilibration and mechanical stability, it does not reach the efficiency of advanced computational annealing protocols such as the Swap Monte Carlo algorithm. The latter employs non-local particle swapping moves that allow the system to access exceedingly low-energy configurations, far beyond reach for local activity-driven rearrangements. Future research will explore whether combining activity with such computational techniques can synergistically push the boundaries of glass equilibration and stability.
Moreover, the study opens intriguing avenues centered on the memory and learning aspects of active matter. Investigating how metabolic control modulates these memory effects could revolutionize our grasp of tissue adaptation and cellular decision-making. The interplay between mechanics, metabolism, and information storage in living materials stands as a frontier ripe for exploration, blending physics, biology, and materials science.
In summary, the work by Sharma and Karmakar sheds light on the fundamental physics of active amorphous solids, offering a fresh paradigm wherein internal activity substitutes for thermal annealing to rigidify materials. By bridging active matter and cyclically driven passive glasses, it not only deepens our theoretical understanding but also lays the foundation for engineering materials capable of self-directed mechanical evolution, akin to living tissues. This research heralds a new chapter in the science of soft condensed matter and bioinspired materials design, with far-reaching implications for technology and medicine.
Subject of Research: Computational simulation/modeling of active glasses and their mechanical annealing
Article Title: Activity-induced annealing leads to a ductile-to-brittle transition in amorphous solids
News Publication Date: 2-Jan-2025
Web References: DOI 10.1038/s41567-024-02724-5
Keywords
Active glasses, amorphous solids, mechanical annealing, ductile-to-brittle transition, energy landscape, oscillatory shear, computational modeling, tissue mechanics, metamaterials, memory effects, Swap Monte Carlo, active matter
Tags: active glassesadaptive behavior in materialsamorphous solids vs crystalline solidsbiological tissue mechanicscomputational simulations in material scienceglass transition temperature effectsinnovative material designinternal energy sources in materialsmechanical properties of glassesself-regulating materialssynthetic materials with tunable propertiesTata Institute of Fundamental Research
What's Your Reaction?
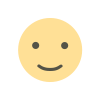
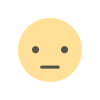
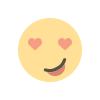
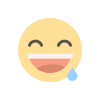
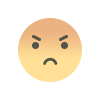
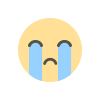
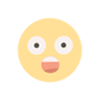