Reconfigurable Nonvolatile Image Processing via Nonlocal Metaoptics
In the rapidly evolving realm of photonics and optical computing, a groundbreaking advancement has emerged that promises to redefine how we manipulate images and information at the fundamental level. A recent study led by Yang, G., Wang, M., Lee, J.S., and colleagues unveils a novel class of nonlocal phase-change metaoptics designed for reconfigurable, nonvolatile image […]

In the rapidly evolving realm of photonics and optical computing, a groundbreaking advancement has emerged that promises to redefine how we manipulate images and information at the fundamental level. A recent study led by Yang, G., Wang, M., Lee, J.S., and colleagues unveils a novel class of nonlocal phase-change metaoptics designed for reconfigurable, nonvolatile image processing. Published in Light: Science & Applications in 2025, this innovative approach combines phase-change materials with metaoptical architectures to achieve unprecedented control over light-matter interaction, opening new horizons in optical technologies.
At the heart of this pioneering development lies the concept of nonlocality within phase-change metaoptics, an area that pushes beyond conventional metasurface functionalities. Unlike traditional metasurfaces, where the response is typically localized and tied to individual meta-atoms, the nonlocal paradigm integrates interactions across multiple meta-elements. This collective behavior enables complex, reconfigurable optical functionalities that can be programmed—and importantly, retained without continuous power input, thus termed “nonvolatile.”
Phase-change materials (PCMs), like the well-known chalcogenide compounds, have long been celebrated in photonics for their capability to swiftly and reversibly switch between amorphous and crystalline states. These states exhibit dramatically different optical properties, such as refractive index and absorption coefficients, lending themselves naturally to dynamic photonic devices. The novel contribution by Yang et al. expounds on these materials’ potential by embedding them within an engineered metaoptic platform that harnesses their phase-transition agility for spatially and temporally programmable image modulation.
One of the most remarkable aspects of this research is the implementation of nonlocality to achieve spatially extended interactions across the metaoptic array. By designing these meta-structures to allow for cooperative coupling, the device transcends the limitations of pixel-by-pixel modulation, enabling the manipulation of optical wavefronts and phase profiles over larger scales internally. This creates the capacity for complex image processing tasks such as reconfiguration, filtering, and encoding, without the need for mechanical components or continuous external control signals.
The practical implications for this technology are vast, touching on fields from augmented reality and holography to neuromorphic computing and optical data storage. Specifically, the ability to reconfigure optical elements in a nonvolatile fashion—meaning the programmed image or optical state remains intact without power—addresses the critical challenge of energy efficiency. This is particularly relevant in scalable image-processing systems where power consumption and device stability are paramount.
Technically, the team fabricated their metaoptic platform by integrating thin films of phase-change material onto nanostructured substrates that had been precisely engineered to facilitate the desired nonlocal interactions. The resultant device exhibited enhanced modulation depths and contrast ratios when switching between different programmable optical states. Remarkably, the switching was reversible and repeatable over numerous cycles, highlighting the robustness of the PCM integration and the metaoptic design.
In addition to the experimental achievements, the researchers developed comprehensive theoretical models to describe the underlying physics governing nonlocal interactions in phase-change metaoptics. These models accounted for the coupling between adjacent meta-elements mediated by both near-field and far-field effects, offering deep insights into how these interactions influence overall device performance. Such theoretical groundwork is essential for guiding future design optimizations and pushing the limits of optical functionality further.
Another dimension of this work was the demonstration of image processing capabilities directly on the metaoptic device. Instead of simply modulating a single parameter, the platform could spatially encode complex images and reconfigure these patterns dynamically through controlled phase transitions. This represents a paradigm shift from static optical components to truly programmable, adaptive photonic systems capable of in-situ image manipulation.
The implications for optical communication networks are also significant. With reconfigurable, high-fidelity metaoptics that operate passively when in a programmed state, one can envision novel routing and signal processing components that minimize power draw while maximizing flexibility and throughput. Furthermore, the enhanced integration of phase-change materials suggests pathways toward all-optical memories and logic elements, further bridging the gap between photonics and computation.
From a materials science perspective, the choice and engineering of phase-change compounds were critical. Ensuring fast switching speeds, high optical contrast, and material stability over thousands of cycles demanded meticulous synthesis and characterization. The study pushes these boundaries by demonstrating that carefully controlled nanostructuring of PCM films can tailor both their optical response and phase-transition dynamics, further enriching the toolkit available to optical designers.
Importantly, the research addresses longstanding challenges associated with integrating PCMs into metasurfaces, such as thermal management and nanoscale fabrication precision. Employing advanced lithographic techniques and innovative layer deposition protocols, the team overcame obstacles that typically impair device yield and performance uniformity. These technical feats underscore the feasibility of scaling such metaoptic systems for practical applications.
Looking toward future prospects, the integration of nonlocal phase-change metaoptics with emerging technologies like machine learning and adaptive control algorithms could accelerate real-time, reconfigurable optical computing platforms. These adaptive metaoptics could form the backbone of next-generation smart optics, capable of perceiving, learning, and reacting to environmental inputs without human intervention.
Moreover, the synergy of nonvolatility and reconfigurability in the metaoptic platform invites cross-disciplinary exploration, including quantum photonics, where dynamic control of light-matter interactions at the nanoscale is critical. The ability to lock in complex phase patterns stably and switch them rapidly lends itself well to quantum information processing and secure communications.
Critically, this advancement also aligns with the growing demand for miniaturization and integration in photonic devices. By enabling multifunctional, programmable metaoptics at subwavelength scales, such technology paves the way for compact, chip-scale optical processors and sensors that outperform traditional electronic counterparts in speed and bandwidth.
As this field matures, one can anticipate a cascade of further innovations spurred by this foundational work. The demonstrated proof-of-concept offers a versatile platform upon which numerous tailored optical functionalities can be built, from dynamic beam shaping and tunable filters to multi-channel optical encryption devices.
In summary, the work by Yang and colleagues represents a monumental stride in the intersection of phase-change materials and metasurface engineering. Their elucidation of nonlocal interactions and integration of nonvolatile reconfigurability marks a new chapter in optical meta-technology, one that holds promise for revolutionizing image processing, photonic computation, and beyond. The lasting impact of this approach will likely reverberate across scientific disciplines and industry sectors, heralding a future where light can be precisely and permanently programmed in complex, multifunctional ways.
Subject of Research: Nonlocal phase-change metaoptics enabling reconfigurable and nonvolatile image processing
Article Title: Nonlocal phase-change metaoptics for reconfigurable nonvolatile image processing
Article References:
Yang, G., Wang, M., Lee, J.S. et al. Nonlocal phase-change metaoptics for reconfigurable nonvolatile image processing. Light Sci Appl 14, 182 (2025). https://doi.org/10.1038/s41377-025-01841-x
Image Credits: AI Generated
DOI: https://doi.org/10.1038/s41377-025-01841-x
Tags: chalcogenide compounds in opticsdynamic photonic devicesimage manipulation techniqueslight-matter interaction controlmetasurfaces and nonlocalitynonlocal phase-change metaopticsoptical computing advancementsoptical technology innovationsphase-change materials in photonicsphotonics research breakthroughsprogrammable optical functionalitiesreconfigurable nonvolatile image processing
What's Your Reaction?
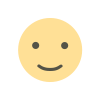
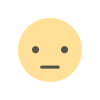
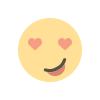
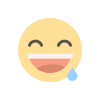
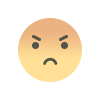
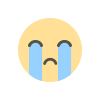
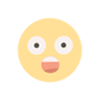