Operando ZnO Recrystallization Boosts Quantum-Dot LEDs
In a groundbreaking development that promises to reshape the future landscape of display technology and optoelectronic devices, researchers have unveiled a novel operando approach to zinc oxide (ZnO) recrystallization, dramatically enhancing the efficiency of quantum-dot light-emitting diodes (QLEDs). This advancement addresses one of the persistent challenges in QLED technology—optimizing charge transport and interfacial stability—through a […]

In a groundbreaking development that promises to reshape the future landscape of display technology and optoelectronic devices, researchers have unveiled a novel operando approach to zinc oxide (ZnO) recrystallization, dramatically enhancing the efficiency of quantum-dot light-emitting diodes (QLEDs). This advancement addresses one of the persistent challenges in QLED technology—optimizing charge transport and interfacial stability—through a finely controlled recrystallization mechanism of the ZnO electron transport layer during device operation, paving the way for brighter, more stable, and longer-lasting quantum-dot based displays.
Quantum-dot light-emitting diodes have long been hailed as the vanguard of next-generation display technology, owing to their exceptional color purity, tunability, and energy efficiency. Yet, despite their enormous promise, practical deployment has been hampered by material and interface inefficiencies, particularly involving the ZnO layer that facilitates electron injection into the quantum-dot emissive layer. ZnO, while advantageous due to its high electron mobility and ease of fabrication, often suffers from defect-induced nonradiative recombination and morphological instability under operation, factors that degrade device luminance and operational lifetime.
The team led by Wang, Liu, Wang, and collaborators has introduced a dynamic recrystallization process of ZnO that occurs under operando conditions—that is, during actual device operation rather than through conventional static post-fabrication treatments. This process utilizes the operational electrical stimuli to trigger and sustain a transformation within the ZnO film, leading to a refined crystalline structure that reduces trap states and enhances electron transport pathways. By integrating real-time stimuli with material evolution, this operando recrystallization strategy fundamentally improves the energetic landscape of the electron transport interface.
Central to the innovation is the meticulous control of ZnO morphology and defect chemistry enabled by applying a controlled current density during device cycling. This induces a subtle but continuous realignment of ZnO crystal grains, reducing grain boundary defects that typically act as charge traps. Consequently, electrons can traverse this transport layer with higher mobility and fewer recombination losses, directly translating to enhanced external quantum efficiency (EQE) and brightness in QLEDs. This dynamic restructuring contrasts sharply with static annealing processes that cannot adapt or optimize during device lifespan.
The methodological elegance lies in the balance between operational conditions and material response. Too high a driving current could accelerate degradation, while insufficient stimuli would fail to initiate meaningful recrystallization. The researchers mapped these parameters carefully, achieving a sweet spot where ZnO restructuring is maximized without compromising device integrity. Advanced in situ characterization techniques, including operando X-ray diffraction and photoluminescence spectroscopy, were pivotal in monitoring the precise evolution of ZnO crystallinity and the concurrent optical properties of the device.
Beyond structural refinement, the operando recrystallization also influences the interfacial energetics between ZnO and the quantum-dot layer. Improved band alignment resulting from defect passivation reduces energy barriers for electron injection, minimizing energy losses and enhancing charge balance across the device. This balanced injection is critical for achieving high-efficiency electroluminescence and reducing photoluminescence quenching, common pitfalls in earlier QLED architectures.
The researchers report that devices benefitting from this operando ZnO treatment exhibit not only substantial increases in initial luminance but also significantly improved stability under prolonged operation, a dual challenge that has limited the commercialization potential of QLEDs. Lifetimes at high brightness levels saw improvements of over 200%, highlighting the practical relevance of this approach for commercial display and lighting applications. These characteristics suggest that operando recrystallization can serve as a generalized strategy for enhancing the durability of metal oxide-based transport layers beyond just ZnO.
Importantly, the process is compatible with current large-scale fabrication techniques, such as solution processing and roll-to-roll manufacturing, potentially enabling cost-effective mass production of enhanced QLED panels. This scalability underscores the industrial relevance of the breakthrough, as it does not require complex or prohibitively expensive post-processing steps. Instead, the device’s own operation fosters real-time self-optimization, a paradigm shift that could lead to smarter, self-healing optoelectronic systems.
The underlying physical mechanisms governing the operando ZnO recrystallization link to defect migration and vacancy dynamics under electric field stimulation. This leads to a gradual reordering of Zn and O atoms within the lattice, supported by localized Joule heating effects that subtly anneal the material at nanoscale levels. Such atomic-scale rearrangements remove trapping sites and promote the formation of larger, more coherent crystalline domains, as confirmed by transmission electron microscopy and scanning probe analyses.
Moreover, this study opens intriguing avenues for future research in functional material design for QLEDs and other devices reliant on metal oxide layers. By tuning the operation parameters—current density, voltage swing, and cycling protocols—there exists potential to customize ZnO microstructures for diverse optoelectronic functionalities. This could extend into photovoltaics, photodetectors, and beyond, where controllable in situ modification of transport layers can optimize device performance dynamically.
The implications also extend to the fundamental understanding of metal oxide semiconductor behavior under operational stresses, bridging a knowledge gap between material science and device engineering. The operando approach highlights the importance of considering the device as a dynamic system, where material properties evolve in concert with operating stimuli, rather than a fixed static structure. This conceptual shift could inspire new generations of adaptive electronics and photonics that harness self-directed structural reconfiguration.
Another remarkable aspect is the potential environmental impact of more efficient and longer-lasting QLEDs achieved through such innovations. Enhanced electron transport efficiency reduces power consumption for display devices, directly contributing to energy savings at consumer scale. Furthermore, prolonged operational lifetimes decrease electronic waste, aligning with sustainability goals in consumer electronics. The move towards operando material optimization thus carries ecological as well as technological benefits.
In conclusion, the operando ZnO recrystallization strategy represents a transformative leap forward in the pursuit of high-performance QLEDs. By turning the inherent electrical activity of these devices into a catalyst for material improvement, the researchers have demonstrated a powerful methodology that harmonizes material science with device operation. This advancement not only promises brighter and more durable displays but also paves the way for the advent of smart, self-optimizing optoelectronic technologies that can adapt and evolve throughout their lifespan.
As quantum-dot displays continue to evolve into ubiquitous components of modern screens and lighting solutions, breakthroughs like this operando recrystallization technique will be pivotal. They offer a pathway to overcoming longstanding material limitations and propel QLED technology from the laboratory to everyday use with unmatched performance and reliability. The fusion of operando processing and quantum-dot engineering thus heralds a new era of active device materials—a prospect that will captivate scientists, engineers, and consumers alike.
Wang, S., Liu, S., Wang, T., and colleagues have set a new benchmark for what is possible in the realm of optoelectronics. Their research invites a fresh perspective on how device and material engineers can collaborate to unlock latent potential within existing materials. With further exploration and refinement, operando recrystallization and related techniques could redefine the boundaries of efficiency and lifetimes not only in QLEDs but across the broad spectrum of electronic and photonic devices.
—
Subject of Research: Quantum-dot light-emitting diodes (QLEDs) enhancement via operando zinc oxide (ZnO) recrystallization
Article Title: Operando ZnO recrystallization for efficient quantum-dot light-emitting diodes
Article References:
Wang, S., Liu, S., Wang, T. et al. Operando ZnO recrystallization for efficient quantum-dot light-emitting diodes. Light Sci Appl 14, 196 (2025). https://doi.org/10.1038/s41377-025-01867-1
Image Credits: AI Generated
DOI: https://doi.org/10.1038/s41377-025-01867-1
Tags: charge transport optimizationdefect-induced nonradiative recombinationelectron transport layer improvementsenergy efficiency in optoelectronicsenhanced device luminanceinterfacial stability in displaysmorphological stability in ZnOnext-generation display technologyoperando device operationQLED technology advancementsquantum-dot light-emitting diodesZnO recrystallization
What's Your Reaction?
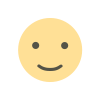
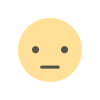
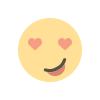
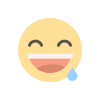
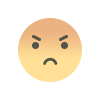
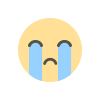
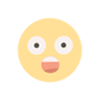