Gyromagnetic Zero-Index Media Reveal Vortex Link
In recent years, the exploration of photonic materials that exhibit extraordinary electromagnetic properties has captivated researchers worldwide. One of the most intriguing developments in this realm is the emergence of photonic double-zero-index media—materials characterized by simultaneously vanishing permittivity and permeability. These unique materials, virtually non-existent in the natural world, offer unprecedented control over light-matter interactions, […]

In recent years, the exploration of photonic materials that exhibit extraordinary electromagnetic properties has captivated researchers worldwide. One of the most intriguing developments in this realm is the emergence of photonic double-zero-index media—materials characterized by simultaneously vanishing permittivity and permeability. These unique materials, virtually non-existent in the natural world, offer unprecedented control over light-matter interactions, heralding a new frontier in photonics. A groundbreaking study now pushes this field further by unveiling a novel class of gyromagnetic double-zero-index metamaterials that marry the extreme parameter regime of zero-index media with the essential non-reciprocal features gleaned from magnetic effects, embedding them within topological frameworks.
Conventional zero-index metamaterials are recognized for their extraordinary ability to “freeze” the phase of electromagnetic waves, enabling phenomena like total transmission or reflection, and facilitating novel applications such as cloaking, beam steering, and enhanced nonlinear interactions. Yet, this paradigm has largely been confined to reciprocal systems with scalar constitutive parameters. By extending the concept to gyromagnetic media, where the permeability and permittivity tensors become non-reciprocal and anisotropic, the researchers have introduced an entirely new dimension of optical behavior, characterized by zero matrix determinants rather than strictly zero scalar values. This subtle yet profound shift enlarges the design space, allowing for metamaterials exhibiting dual zero-index characteristics combined with robust direction-dependent optical responses.
Central to this advance is the identification and experimental realization of a spin-1/2 Dirac point within the metamaterial’s band structure. Such Dirac points act as critical junctures of topological phase transitions, where the material’s electromagnetic properties undergo qualitative transformation. Unlike traditional analyses concentrating solely on spatial topological boundary effects, this work fundamentally links the bulk topological invariants with emergent phenomena in the spatiotemporal domain. The researchers discover that the Dirac point anchors a spatiotemporal reflection vortex singularity—a topological defect in the evolution of the reflected electromagnetic field when both space and time variations are considered. This vortex possesses a quantized charge directly tied to the leap in the system’s topological invariants across the phase transition, forging a new bulk–spatiotemporal vortex correspondence paradigm.
This novel correspondence transcends established principles in topological photonics, which typically connect bulk topology with edge states or boundary modes localized in space. By extending the topological characterization into the time domain, the research ushers in a new class of bulk-boundary type dualities that control the temporal dynamics of light reflected or transmitted through the metamaterial. This is not just a theoretical curiosity; the existence of such spatiotemporal vortices signifies a new handle on manipulating the waveform, phase singularities, and temporal coherence of light at extreme parameter regimes, enabling previously unattainable control over optical fields.
To unlock the practical potential of this discovery, the team innovated a mechanism to deterministically generate optical spatiotemporal vortex pulses anchored at the Dirac point. These vortex pulses maintain firmly fixed central frequency and momentum, directly imposed by the topological origin of their formation. This rigidity confers ultra-robustness to the pulses, making them resilient against structural imperfections, environmental fluctuations, and perturbations that would normally degrade coherence or entangle phase singularities. The ability to reliably produce such pulses paves the way for cutting-edge applications in optical communications, quantum information processing, and advanced imaging technologies where stability and precision of light-matter interaction are paramount.
Methodologically, achieving the gyromagnetic double-zero-index conditions requires precise engineering of both the permittivity and permeability tensors, exploiting accidental degeneracies of photonic bands to generate Dirac cones within the material’s dispersion relation. Historically, Dirac cones in photonic crystals have been associated with zero-index behavior due to their linear dispersion and associated zero effective refractive index at the cone point. By integrating magneto-optical effects breaking time-reversal symmetry, the research ensures non-reciprocity and a rich topological phase diagram wherein the spin-1/2 nature of the Dirac point emerges naturally.
The experimental realization was validated through advanced fabrication techniques and comprehensive electromagnetic characterization, confirming the presence of the topological phase transition and its associated spatiotemporal vortex singularity. Reflection measurements analyzed in the combined space-time Fourier domain revealed phase singularities winding with quantized topological charges, directly linked to the theoretical vortices predicted. Such corroboration highlights the seamless interplay between theoretical photonics, material engineering, and experimental optical physics, underpinning the robustness of the conclusions.
Beyond its immediate impact on zero-index photonics, this research stands at the intersection of several vibrant fields including topological photonics and singular optics. By revealing topological phase-transition points as intrinsic generators of spatiotemporal vortex singularities, it suggests a new approach to manipulate complex light fields using engineered metamaterial topologies. This convergence creates myriad possibilities—for example, enabling topological control over ultrafast pulses, harnessing singular optical waveforms to encode information robustly, and enhancing nonlinear light-matter interactions through topologically-protected modes.
Moreover, the study challenges traditional paradigms where spatial topology alone dictates the behavior of photonic devices, advocating instead for a holistic approach that incorporates temporal topological phenomena. Such an approach might stimulate innovations in dynamic photonic systems, where space and time are intricately entwined in shaping wave propagation. Future devices could exploit this to dynamically reconfigure their optical response or to produce temporally structured light fields with unprecedented resilience against noise and disorder.
The discovery also invites new theoretical explorations. The connection between spin-1/2 Dirac points, topological invariants, and spatiotemporal vortices could be extended to other wave systems beyond photonics, including acoustics, plasmonics, and even matter waves. The universality of zero-index and topological concepts implies these principles may be leveraged across a broad spectrum of physical platforms, yielding cross-disciplinary innovations and a deeper understanding of wave physics at extreme limits.
Crucially, the work reinforces the concept that metamaterials with extreme electromagnetic parameters are not mere curiosities but fertile ground for discovering new physics and functionalities. The intricate interplay between double-zero conditions, non-reciprocity, and topology introduces design strategies that can enhance device performance and enable new modes of operation unavailable in conventional optics.
In sum, the experimental realization of gyromagnetic double-zero-index metamaterials accompanied by an intrinsic bulk–spatiotemporal vortex correspondence marks a paradigm shift. It illuminates topological phase-transition points as dynamic generators of stable spatiotemporal singularities, unlocking new avenues to harness light in time and space. The robust generation of spatiotemporal vortex pulses with fixed frequencies and momenta epitomizes the power of integrating topology, magneto-optical effects, and metamaterial design. As these insights permeate the broader photonics community, they will invariably catalyze breakthroughs in optical technologies and inspire the next generation of photonic devices that command light with topological precision.
Subject of Research: Gyromagnetic double-zero-index photonic metamaterials and their topological spatiotemporal vortex singularities during phase transitions.
Article Title: Bulk–spatiotemporal vortex correspondence in gyromagnetic zero-index media
Article References:
Zhang, RY., Cui, X., Zeng, YS. et al. Bulk–spatiotemporal vortex correspondence in gyromagnetic zero-index media. Nature (2025). https://doi.org/10.1038/s41586-025-08948-6
Image Credits: AI Generated
Tags: anisotropic permittivity and permeabilityapplications of zero-index mediacloaking and beam steering technologieselectromagnetic wave phase freezingenhanced nonlinear interactions in photonicsgyromagnetic double-zero-index metamaterialsinnovative designs in photonic materialslight-matter interactions in photonicsnon-reciprocal electromagnetic interactionsphotonic materials with extraordinary propertiestopological frameworks in metamaterialsunconventional optical behavior in gyromagnetic media
What's Your Reaction?
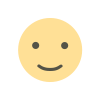
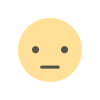
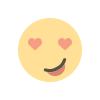
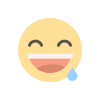
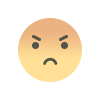
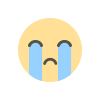
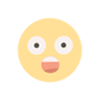