Non-Coding RNA: Key Players in Protein Synthesis and Cellular Stress Response
In a groundbreaking study, researchers at The Ohio State University have made significant strides in understanding the role of certain RNA segments that were traditionally categorized as non-functional or “junk” DNA. This research uncovers the functional capacities of these segments, specifically focusing on introns that are part of transfer RNA (tRNA). The study reveals that […]

In a groundbreaking study, researchers at The Ohio State University have made significant strides in understanding the role of certain RNA segments that were traditionally categorized as non-functional or “junk” DNA. This research uncovers the functional capacities of these segments, specifically focusing on introns that are part of transfer RNA (tRNA). The study reveals that these introns possess the ability to suppress messenger RNA (mRNA) production, thus aiding cells in managing responses to oxidative stress—an important finding that challenges long-standing beliefs about RNA functionality.
RNA, or ribonucleic acid, is a vital component in the process of translating genetic information into proteins. Within this complex paradigm, tRNA serves a pivotal role by carrying amino acids to the ribosome, the site of protein synthesis. Traditionally, introns—non-coding regions found within the tRNA—have been relegated to the status of evolutionary relics, with little thought given to their potential utility. However, this recent research, published in the prestigious journal Molecular Cell, illuminates a novel function for these small segments that may well redefine our understanding of cellular biology.
Anita Hopper, the senior author of the study, spearheaded an investigation into what researchers have termed “fitRNAs,” short for free introns of tRNAs. These fitRNAs are not merely leftover fragments; they engage actively with mRNA molecules responsible for coding proteins. Through a series of meticulously designed experiments, the research team observed that the attachments made by these fitRNAs can precipitate the degradation of target mRNAs. This indicates that fitRNAs may serve as a regulatory mechanism that effectively halts protein production when the cellular environment becomes stressed.
The implications of this study are profound. In the past, the prevailing perspective was that cells expended energy in eliminating introns due to their perceived lack of usefulness. Yet, the researchers encountered an alternative narrative: the selective destruction mechanisms employed by cells to manage these introns suggested that they may indeed have an operative role. This conundrum led to a deeper inquiry into the evolutionary significance of these sequences, prompting the team to undertake a rigorous exploration of their stability and interactions with other RNA types under varying conditions.
In particular, when cells were subjected to oxidative stress—an imbalance between free radicals and antioxidants in the body—one type of intron exhibited remarkable stability. This resilience hints at the possibility that these segments not only contribute to the evolutionary toolbox of the cell but also provide adaptive advantages during stressful times. It raises the intriguing question of why such segments would persist across various organisms if they were truly redundant or inefficient, suggesting an underlying importance that had been overlooked for decades.
The study employed yeast as a model organism, a strategic choice due to its simplicity and the wealth of genetic tools available for interrogating RNA behavior. The initial exploration of introns in tRNA has now evolved into a broader inquiry about their potential interactions and roles across different species, from humans to mice and even insects. This opens a new chapter in our understanding of gene regulation, one that highlights an intersection where evolution meets molecular biology.
Hopper’s team focused their research on two particular intron families, each showcasing unique interactions and decay mechanisms. The findings confirm that once the tRNA is processed and the introns are released, they can bind complementary sequences on mRNA molecules. This engagement leads to targeted mRNA degradation, which effectively silences the gene’s expression. Thus, the introns serve not just as relics, but as active regulators capable of making significant impacts on cellular function.
Moreover, while the functionality of these fitRNAs bears resemblance to that of microRNAs—small RNA molecules known to play critical roles in gene expression regulation—there are key differences in their operational mechanics. MicroRNAs typically require protein partners such as Argonaute to facilitate mRNA degradation. In contrast, the research found that fitRNAs operate independently of Argonaute proteins in yeast, demonstrating a different approach to gene regulation.
What remains particularly compelling about this study is the broader question of why fitRNAs stabilize during oxidative stress. As cells navigate challenging environments—be it through oxidative stress, starvation, or heat stress—these introns could serve as negative regulators of gene expression, offering a strategic means for cells to conserve resources and prioritize vital functions. This adaptability provides a glimpse into the sophisticated mechanisms by which cells maintain homeostasis in the face of environmental challenges.
Expanding on these insights, Paolo Sinopoli, one of the co-authors of the study, highlights that the presence of introns across various life forms suggests an evolutionary endurance and functional relevance beyond mere byproducts of genetic machinery. The researchers identified a multitude of mRNAs targeted by intron segments, which primarily affect proteins associated with critical processes such as cell division and reproduction. This connection unveils the potential transformative role these introns might play in the landscape of molecular genetics.
The researchers are particularly interested in further exploring the equilibrium between intron stability and mRNA degradation under different forms of cellular stress. Understanding this balance will be crucial in deciphering how cells adapt to their environments and may lead to further elucidations of cellular regulation mechanisms that have profound implications in health and disease.
Thus, as this research establishes a framework for reevaluating the importance of introns, it not only reveals the complexity of gene expression regulation but also highlights the evolutionary ingenuity of cellular mechanisms. The findings pave the way for new explorations into the vast, often-overlooked world of RNA biology, offering exciting possibilities for potential applications in health and disease management.
This research exemplifies the dynamic nature of science, demonstrating how what was once deemed trivial can evolve into a cornerstone of our understanding of cellular regulation. As science continues to progress, it beckons us to reconsider our assumptions and to remain open to the unexpected, especially in realms as intricate and pivotal as molecular genetics.
Subject of Research: Free introns of tRNAs and their role in gene expression regulation
Article Title: Free introns of tRNAs as complementarity-dependent regulators of gene expression
News Publication Date: 11-Feb-2025
Web References: Molecular Cell
References: Not available
Image Credits: Not available
Keywords: RNA, introns, fitRNAs, gene expression, oxidative stress, mRNA degradation, tRNA, molecular genetics, Ohio State University, evolutionary biology.
Tags: cellular stress managementevolutionary significance of intronsfitRNAs and their significanceimplications for genetic researchintrons in transfer RNAmessenger RNA suppressionmolecular biology breakthroughsnon-coding RNA functionalityOhio State University researchoxidative stress response in cellsprotein synthesis mechanismsrole of tRNA in protein production
What's Your Reaction?
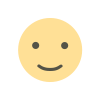
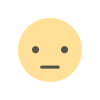
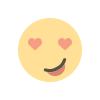
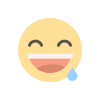
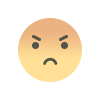
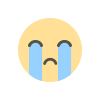
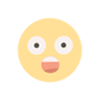