Modular Chiral Origami Transforms Metamaterials Design
In the rapidly evolving landscape of materials science, the emergence of metamaterials with intricate deformation mechanisms is reshaping how engineers and physicists conceive machine-like behavior in synthetic structures. These engineered materials mimic mechanical machines by harnessing multimodal actuation, allowing unprecedented control over their responses to external stimuli. A groundbreaking study by Zhao et al., published […]

In the rapidly evolving landscape of materials science, the emergence of metamaterials with intricate deformation mechanisms is reshaping how engineers and physicists conceive machine-like behavior in synthetic structures. These engineered materials mimic mechanical machines by harnessing multimodal actuation, allowing unprecedented control over their responses to external stimuli. A groundbreaking study by Zhao et al., published recently in Nature, delivers an innovative leap in this domain by introducing modular chiral origami metamaterials capable of decoupled, large-scale deformation and versatile functionalities.
Traditional chiral metamaterials, characterized by their ability to convert linear inputs into rotational outputs, have garnered attention for their intrinsic dual-modality. These structures exhibit unique properties such as enhanced wave manipulation, circular polarization control, and applications in chiral active fluids. However, their practical deployment has long been stymied by two critical limitations: the coupling of deformation modes prevents independent control over twisting and contraction, and their allowable strain typically fails to exceed 2%, restricting their operational range.
Zhao and colleagues circumvent these barriers by conceptualizing and fabricating a modular assembly that combines auxetic planar tessellations with origami-inspired columnar arrays, engineering a platform where in-plane and out-of-plane deformations can be selectively actuated. The metamaterial is designed to operate under a single-degree-of-freedom actuation scheme, prompting the assembly to twist through a broad 0° to 90° range, contract in-plane by as much as 25%, and shrink out-of-plane by over 50%. This decoupling of motion control is a significant deviation from prior metamaterial systems and sets new standards for adaptability.
At the heart of this metamaterial’s performance is the ingenious integration of rotating-square tessellations and tubular Kresling origami arrays. The planar tessellations predominantly govern in-plane twist and contraction, leveraging the auxetic behavior to achieve a controlled and reversible deformation. Simultaneously, the tubular Kresling columns provide robust out-of-plane shrinkage through their origami-dependent bistability and folding transitions. This bifurcation of roles allows for precise, independent manipulation of complex shape changes.
The researchers employed a blend of experimental prototyping and computational simulations to probe the mechanical responses of these assemblies. Physical models fabricated using additive manufacturing techniques confirmed the theoretical predictions, showcasing smooth transitions between twisted and contracted states as well as dramatic vertical compression without loss of structural integrity. Numerical models further illuminated the stress distributions and energy landscapes underpinning the modular metamaterial’s remarkable ability to switch deformation modes selectively.
One of the most remarkable achievements presented in the study is the identification of two distinct actuation regimes. The first regime enables twisting of the metamaterial with unconstrained translational movement, maintaining the modular assembly’s spatial positioning. The second allows linear displacement along the vertical axis with free rotation, facilitating versatile mechanical reconfigurations. These regimes highlight an unprecedented level of tunability and suggest potential for deployment in adaptive robotics and responsive architecture.
The modularity of the assembly is a critical enabler of its multifunctionality. By connecting discrete units of tessellations and origami arrays, the architecture supports reprogrammable mechanical instability, a feature where the metamaterial’s deformation pathway can be adjusted post-fabrication. This adaptability extends to local chirality control, allowing selective tuning of handedness within the material, a feature rarely achievable in bulk-engineered chiral metamaterials.
Beyond mechanical performance, the metamaterial exhibits scalable load-bearing capabilities, making it suitable for applications stretching from micro-scale devices to large-scale engineering structures. Its capacity for multistability—the presence of multiple stable deformation states—introduces opportunities for mechanical memories encoded within hysteresis loops, enabling systems that retain deformation states without continuous applied forces.
The implications of this modular chiral origami metamaterial reverberate across multiple scientific and engineering fields. Robotic transformers, capable of reconfiguring their shape and motion modes autonomously, stand to benefit considerably. Similarly, thermoregulation systems could exploit the metamaterial’s large, reversible volume changes to modulate thermal conductivity or airflow dynamically.
Another fascinating avenue opened by this work is the potential for implementing non-commutative state transitions within mechanical systems—where the order of deformation operations affects the final state, enabling complex information processing behaviors. This could lead to mechanical computing elements that function based on physically encoded sequences, harnessing the metamaterial’s multistability and modular kinetics.
Furthermore, the plug-and-play nature of the metamaterial’s design offers facile integration into energy-absorbing devices, where impact dissipation can be tuned by activating specific deformation modes. Applications in information encryption are also conceivable, as spatially patterned chirality and multistable states can encode data within physical configurations resistant to trivial decoding.
In essence, Zhao et al. present a new class of architected materials that bridge the gap between static metamaterials and dynamic, machine-like systems. Their work showcases the power of combining classical auxetic principles with origami-inspired design, yielding metamaterials that are not only mechanically versatile but also programmable and scalable.
The study propels the paradigm of metamaterials towards becoming true mechanical ‘machines’ capable of adaptive, autonomous function. It underscores a future where materials themselves perform complex tasks traditionally reserved for engineered devices, opening a vista of transformative applications tied to multimodal deformation, active function, and reprogrammability.
As the relentless advancement of metamaterial science continues, this research marks a pivotal moment where chiral origami techniques and auxetic assemblies converge to redefine the mechanical intelligence embedded within synthetic solids. From robotic actuation to information security, the horizons for these modular chiral systems are expanding, inviting interdisciplinary innovation and experimentation.
The exploration of instability reprogramming, local chirality tuning, multistability, and the integration of foldable architectures warrants further investigation, promising a treasure trove of mechanical functionalities that blend physics, geometry, and material science. The work of Zhao et al. thus stands as a beacon for next-generation metamaterial design, opening avenues for intelligent structures with tailored, tunable, and transformative capabilities.
Subject of Research: Modular chiral origami metamaterials with decoupled multimodal deformation mechanisms enabling large, programmable actuation and multifunctionality.
Article Title: Modular chiral origami metamaterials.
Article References:
Zhao, T., Dang, X., Manos, K. et al. Modular chiral origami metamaterials. Nature 640, 931–940 (2025). https://doi.org/10.1038/s41586-025-08851-0
Image Credits: AI Generated
DOI: https://doi.org/10.1038/s41586-025-08851-0
Keywords: Metamaterials, chiral origami, auxetic tessellations, modular assembly, multimodal deformation, multistability, mechanical memory, programmable instability, robotic transformers, tunable chirality, energy absorption, information encryption
Tags: advancements in materials science researchauxetic planar tessellations in engineeringchiral active fluids applicationsdecoupled large-scale deformationenhanced wave manipulation in metamaterialsindependent control of deformation modeslimitations of traditional chiral metamaterialsmetamaterials design innovationsmodular chiral origami metamaterialsmultimodal actuation in materialsorigami-inspired materials engineeringsynthetic structures with machine-like behavior
What's Your Reaction?
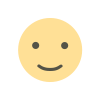
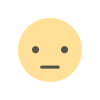
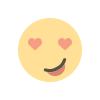
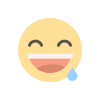
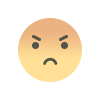
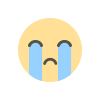
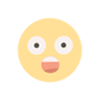