Exploring the Impact of Directional Connections on Complex Dynamics in Neuronal Networks
In a groundbreaking study conducted by researchers at Tohoku University, a significant advancement has been made in understanding how the directional connections within neuronal networks influence their complex dynamics. This research tackles one of the fundamental challenges in biology: deciphering the intricate relationship between neural structure and function. Traditionally, studying these interactions in live animal […]

In a groundbreaking study conducted by researchers at Tohoku University, a significant advancement has been made in understanding how the directional connections within neuronal networks influence their complex dynamics. This research tackles one of the fundamental challenges in biology: deciphering the intricate relationship between neural structure and function. Traditionally, studying these interactions in live animal brains poses substantial challenges due to their complexity, and the invasive nature of the required surgical interventions. This innovative investigation, however, leverages lab-grown neurons with precisely controlled connectivity, providing researchers with a promising alternative to conventional animal testing.
By utilizing microfluidic devices adept at forming directional connections among neurons, the Tohoku University team aimed to explore how these connections affect neuronal activities and the network’s overall behavior. Microfluidics is a leading-edge technology that allows manipulation at the microscale, which was crucial in fabricating neuronal networks that mimic the organization found in living systems. In their experiments, researchers observed how the flow of signals, akin to a downstream river current, was influenced by the presence of these one-way connections, yielding fresh insights into how neural networks process and transmit information.
Directing the flow of neural signals through the strategic design of microchannels offers researchers a unique opportunity: they can now study specific network configurations that align more closely with natural, in-vivo environments. This meticulous design allowed for the fabrication of neurons capable of exhibiting adaptive responses similar to those observed in live animal brains, ultimately enhancing the reliability and applicability of in-vitro models in neuroscience research. Through calcium imaging techniques, the team diligently recorded spontaneous activities exhibited by their fabricated neuronal networks, revealing that networks incorporating directional connections displayed more intricate activity patterns than their non-directional counterparts.
The research’s implications extend beyond understanding basic neural dynamics. For instance, the mathematical models developed by the team were instrumental in predicting how specific configurations and connections could foster complex dynamics within neuronal systems. These models were based on experimental data obtained from their lab-grown networks and provided new frameworks for analyzing the relationship between neuroanatomy and network behavior. By simulating various connection configurations and observing their dynamic output, researchers discovered that integrating modularity within the network was paramount in fostering more sophisticated operational patterns.
This study is a significant stride toward elucidating the enigmatic mechanisms governing brain functionality. Lead author Nobuaki Monma emphasized the brain’s inherent complexity, stating that it continuously adapts and alters its responses to similar stimuli based on numerous factors. The study’s outcomes not only enhance our fundamental comprehension of neuronal networks but also propose that these findings could cascade into various applications across multiple fields, including medicine, artificial intelligence, and machine learning.
Moreover, the development of a robust in-vitro model of biologically plausible artificial neural networks represents a pioneering step towards more accurate and effective systems in artificial intelligence. These insights could lead to the creation of artificial neural networks that replicate biological behaviors more accurately, thus enabling better adaptability and learning akin to their biological counterparts. As the team continues to unravel these complex relationships, advances in theoretical modeling are expected to contribute to analyzing and interpreting larger-scale neural networks, potentially driving forward the ambitious goal of a comprehensive connectome analysis of the brain.
Furthermore, the researchers highlight that understanding these neuronal connections may eventually unlock new avenues in understanding neurodevelopmental disorders and could foster advancements in therapeutic interventions. By bridging the gap between theoretical understanding and practical applications, this research lays foundational work that may lead to enhanced diagnostic and therapeutic strategies for conditions that emanate from neuronal dysfunction.
This landmark study not only provides robust insights into the dynamic interplay of neuronal connections but also sets the stage for exploring new frontiers in neuroscience. As researchers continue to delve into the subtleties of neuronal activity and connectivity, the hope is that this progress will offer profound contributions to our holistic understanding of the brain. The implications of this research are significant, revealing pathways through which neuronal networks might be manipulated or changed, thus opening discussions for potential new treatments for neurological diseases.
With refined insights into the structural and functional correlations in neuronal networks, future research endeavors could focus on tailoring network configurations for specific therapeutic outcomes. Exploring the capacity for these lab-grown neurons to adapt over time offers a promising outlet for research into neuroplasticity and learning mechanisms—core principles that underpin cognitive functions and behavioral adaptability in living organisms.
As neuroscience continues to evolve and merge with engineering principles, the interdisciplinary collaboration between researchers from various fields becomes ever more crucial. The findings from this study underscore the importance of integrating diverse methodologies, offering a more complete understanding of the relationship between structure and function in the brain’s neural architecture. Such collaborative efforts stand to catalyze future innovations and pave the way for enriched exploration into the depths of neural connectivity and its ramifications.
In conclusion, this research not only enhances our fundamental understanding of neuronal networks but also proposes an exciting future where lab-grown models can be utilized to simulate complex brain dynamics. As scientific inquiry progresses, the quest for knowledge surrounding neuronal structure-function relationships will undoubtedly continue to captivate and drive innovation in both basic and applied sciences.
Subject of Research: The role of directional connections in neuronal networks and their impact on dynamic behavior
Article Title: How do Directional Connections Shape Complex Dynamics in Neuronal Networks?
News Publication Date: 28-Nov-2024
Web References: DOI link
References: Neural Networks, November 28, 2024.
Image Credits: ©Nobuaki Monma et al.
Keywords
Neural networks, Anisotropy, Mathematical modeling, Artificial neural networks, Neural modeling, Neurons, Microfluidics, Axons, Bioengineering, Computational neuroscience.
What's Your Reaction?
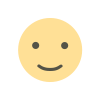
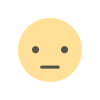
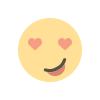
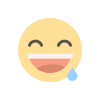
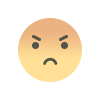
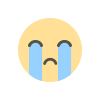
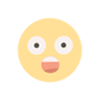