Boosting Mitochondrial DNA Editing via Base Repair
In the rapidly evolving landscape of genetic engineering, the precision editing of mitochondrial DNA (mtDNA) has long presented a formidable challenge to scientists. Mitochondria, the energy-producing organelles within cells, possess their own distinct DNA, and mutations in this genome are linked to a broad spectrum of debilitating diseases. The advent of transcription activator-like effector-linked deaminases […]

In the rapidly evolving landscape of genetic engineering, the precision editing of mitochondrial DNA (mtDNA) has long presented a formidable challenge to scientists. Mitochondria, the energy-producing organelles within cells, possess their own distinct DNA, and mutations in this genome are linked to a broad spectrum of debilitating diseases. The advent of transcription activator-like effector-linked deaminases (TALEDs) has marked a significant step forward in targeting mtDNA, particularly for A-to-G base editing. However, the intricacies underlying their mode of action remained elusive—until now.
Recent groundbreaking research has uncovered the molecular mechanism by which TALEDs harness the cell’s innate DNA repair machinery to enable efficient adenosine-to-guanosine (A-to-G) editing in mitochondrial genomes. By strategically leveraging the base excision repair (BER) pathway, researchers have unlocked a new frontier in precise mitochondrial genome editing. This conceptual breakthrough elucidates how TALEDs convert their substrate DNA into a single-stranded configuration amenable to site-specific adenosine deamination, fundamentally enhancing editing efficiency and specificity.
TALEDs operate through a remarkable fusion of two enzymatic functionalities: the single-stranded DNA-specific adenosine deaminase TadA8e and the double-stranded DNA (dsDNA)-specific cytidine deaminase DddA. While TadA8e mediates the actual A-to-G conversion, the newly revealed secret lies in DddA’s ability to initiate strand-specific modifications. Upon DddA-mediated deamination of cytidine residues, uracil bases arise transiently in the mtDNA, effectively flagging sites for the BER machinery. This initiates a cascade in which the base excision repair system actively excises these uracils, unwinding and transiently converting the surrounding dsDNA into single-stranded DNA (ssDNA) at targeted loci.
In this newly single-stranded state, the DNA becomes accessible to TadA8e, which then executes the adenine-to-inosine deamination, ultimately read as guanine post-replication. This remarkable choreography between cytidine deamination, uracil excision, and single-strand exposure forms the mechanistic foundation for TALED-mediated base editing. Consequently, this study debunks prior assumptions that TALEDs directly bind and edit dsDNA, highlighting instead a critical dependence on the BER pathway for the transient creation of their substrate.
The implications of this discovery have profound practical outcomes. By substituting the native DddA with an engineered, high-activity variant named DddA6, scientists have successfully amplified the initial cytidine deamination step, generating more robust signals for base excision repair engagement. This results in increased formation of single-stranded regions and consequently enhances the overall frequency and efficiency of adenine editing by TadA8e. Complementing this strategy, the fusion of human uracil DNA glycosylase to the TadA8e domain further streamlines uracil recognition and excision, thus fine-tuning the BER activation to optimize the exposure of editing substrates.
The engineering advancements culminated in the creation of a suite of enhanced TALEDs (eTALED6s), which display superior editing kinetics and substantively elevate on-target editing rates in mitochondrial DNA. Yet, the story does not end at sheer efficiency. Advanced iterations, such as the eTALED6R variant, showcase remarkable control over editing precision by minimizing both bystander and off-target modifications in DNA and RNA pools. This balancing act is critical, as uncontrolled editing could compromise mitochondrial function or incur unintended mutagenesis, potentially exacerbating disease phenotypes rather than alleviating them.
These newly engineered TALEDs open avenues for sophisticated mitochondrial gene therapy, enabling the targeted installation or correction of pathogenic point mutations responsible for mitochondrial disorders. This therapeutic promise was demonstrated conclusively through experiments that employed eTALED6 and eTALED6R to successfully introduce a disease-related mutation into mitochondrial genomes, underscoring the potential for both modeling mitochondrial diseases and devising corrective interventions.
The mechanistic insights and protein engineering strategies developed through this work represent an elegant convergence of basic molecular biology and applied biotechnological innovation. By revealing that effective A-to-G editing requires the active recruitment of cellular base excision repair pathways, the study provides a rational framework to design next-generation mitochondrial base editors. This framework can be exploited to improve targeting accuracy, reduce off-target effects, and ultimately expand the toolkit available for mtDNA manipulation.
Furthermore, the study highlights the subtle interplay between DNA damage repair and base editing, illustrating that intentional genomic modification depends not only on catalytic activity but also on the orchestration of endogenous enzymatic environments. Understanding these interdependencies is critical when considering mitochondrial DNA, given its unique architecture, replication dynamics, and limited repair options compared to nuclear DNA.
This work also raises exciting questions about the limits and possibilities of mitochondrial genome editing. For example, could further manipulation of mitochondrial BER components or engineering of additional enzymatic partners enhance editing windows or enable other types of base conversions? Could these insights be extended to other organelles or DNA contexts that are currently refractory to precise modification? Such questions open thriving territory for future exploration.
On a broader scale, by facilitating precise manipulation of mitochondrial genomes, these advances contribute to deciphering the enigmatic role of mtDNA mutations in aging and complex metabolic diseases. Researchers can now investigate causality more accurately by introducing or correcting mutations in living cells or animal models, enabling functional studies unattainable with previous technology. This ability may shed light on mitochondrial contributions to neurodegenerative diseases, cancer metabolism, and inherited metabolic syndromes.
In conclusion, the unraveling of TALED function through a base excision repair-dependent mechanism represents a milestone in mitochondrial genome engineering. The combination of enhanced deaminase variants, fusion proteins targeting uracil excision, and precise spatiotemporal control of DNA substrate availability converges to produce highly efficient and specific mitochondrial base editors. These innovative tools are poised to propel mitochondrial genetics into a new era of precision medicine, promising novel treatments for mitochondrial disorders that have long eluded effective intervention.
This breakthrough also exemplifies the power of dissecting cellular repair pathways to refine genome editing technologies, a principle that may catalyze similar improvements across diverse genome editing platforms. As the field moves forward, the integration of repair biology and protein engineering stands as a paradigm for the development of next-generation therapeutics designed to navigate and manipulate the complexities of human genetics with unprecedented fidelity.
Subject of Research:
Mitochondrial DNA adenine base editing mediated by transcription activator-like effector-linked deaminases and the role of base excision repair.
Article Title:
Leveraging base excision repair for efficient adenine base editing of mitochondrial DNA.
Article References:
Fan, Y., Xu, W., Gao, BQ. et al. Leveraging base excision repair for efficient adenine base editing of mitochondrial DNA. Nat Biotechnol (2025). https://doi.org/10.1038/s41587-025-02608-w
Image Credits: AI Generated
Tags: A-to-G base editing techniquesadenosine-to-guanosine conversionadvancements in mitochondrial genetic therapiesbase excision repair pathwayDNA repair mechanisms in cellsenzymatic functionalities in gene editingmitochondrial diseases and geneticsmitochondrial DNA editingmitochondrial genome mutationsprecision editing of mtDNAsingle-stranded DNA modificationsTALEDs in genetic engineering
What's Your Reaction?
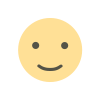
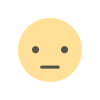
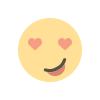
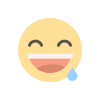
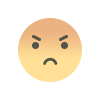
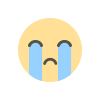
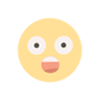