Agile Underwater Vehicles Inspired by Golf Ball Design: Nimble Dimples Make a Splash
In a groundbreaking advancement poised to revolutionize the design and efficiency of underwater and aerial vehicles, researchers at the University of Michigan have unveiled a prototype inspired by an unlikely source: the dimpled surface of a golf ball. This innovative development taps into the profound aerodynamic benefits that golf balls enjoy due to their textured […]

In a groundbreaking advancement poised to revolutionize the design and efficiency of underwater and aerial vehicles, researchers at the University of Michigan have unveiled a prototype inspired by an unlikely source: the dimpled surface of a golf ball. This innovative development taps into the profound aerodynamic benefits that golf balls enjoy due to their textured surface, adapting this principle to dynamically manipulate drag and lift forces on spherical vehicles. By implementing a “smart” morphable skin capable of altering its surface texture in real time, this technology holds the promise of drastically enhancing maneuverability and fuel efficiency in domains ranging from oceanic exploration to airborne robotics.
The genesis of this research lies in an age-old sporting mystery: why do dimpled golf balls travel farther and with more stability than their smooth counterparts? The crux of this lies in the reduction of pressure drag, which is a resistive force that objects encounter when moving through fluid environments such as air or water. Dimples on a golf ball create turbulent boundary layers that cling to the ball’s surface longer, reducing the wake size behind it and, consequently, the pressure drag. This aerodynamic optimization enables golf balls to achieve approximately 30% greater range. Drawing from this insight, the Michigan team engineered a spherical prototype whose surface dimples could be modulated on demand, enabling them to finely tune the interaction between the vehicle’s outer skin and the surrounding fluid.
The heart of the prototype consists of a hollow sphere covered by a thin latex membrane stretched taut over its frame, dotted with an array of minuscule holes through which vacuum pressure can be applied. By selectively activating a vacuum pump, the interior pressure is lowered, forcing the latex inward to create precise dimples of varying depths on its external surface. Crucially, the dimple texture is not static; it can be dynamically adjusted and reversed simply by switching the vacuum system on or off. This level of control over surface morphology creates a powerful platform for studying and exploiting aerodynamic phenomena in a manner that conventional rigid bodies cannot replicate.
To quantitatively assess the impact of dimple modulation on fluid dynamics, the team subjected their prototype to rigorous testing in a 3-meter wind tunnel equipped with sensors and high-speed imaging tools. The spherical vehicle was suspended within the tunnel and exposed to a spectrum of airflow velocities, simulating diverse environmental conditions. Drag forces were measured precisely using a load cell that captured the forces air exerted on the sphere’s surface. Complementary flow visualization techniques employed aerosol tracers coupled with laser illumination, enabling the researchers to observe particle trajectories and turbulent structures as they interacted with the morphable skin in real time.
Results from these experiments revealed a nuanced relationship between dimple depth and drag reduction dependent on flow velocity. At higher wind speeds, shallower dimples proved more effective in disrupting laminar flow separation and mitigating drag, whereas at lower speeds, deeper dimples offered superior performance. Fine-tuning the dimple depth across different conditions consistently allowed the spherical prototype to achieve drag reductions up to an impressive 50% compared to a smooth sphere under identical parameters. This adaptability is vital for real-world applications, where flow conditions are rarely constant and vehicles must optimize performance on the fly.
Beyond drag reduction, the morphable skin technology demonstrated an extraordinary capacity to generate lift forces by exploiting asymmetric surface textures. The researchers devised an inner structural framework designed to induce dimpling on only one hemisphere of the sphere while leaving the opposite side smooth. This asymmetric configuration led to uneven flow separation around the sphere, causing the wake—the turbulent air downstream—to deflect toward the smooth side. According to Newton’s third law, this deviation generates an equal and opposite force pushing the sphere toward the dimpled side, effectively allowing the vehicle to steer by changing the side on which dimples are activated.
Testing this asymmetric morphing revealed lift forces reaching up to 80% of the sphere’s experienced drag force, a remarkable figure for a passive flow control mechanism. These lift magnitudes rival the well-known Magnus effect, where spinning objects generate lift through rotation. However, contrasting with the Magnus effect’s reliance on continuous angular momentum, the morphable skin produces directional control solely by modifying surface roughness—a feat that simplifies design and reduces energy expenditure. Such precise steering capability could eliminate the need for multiple propellers, rudders, or fins typically required by underwater drones and other maneuverable vehicles.
The implications for maritime and aerial technology are profound. Compact spherical submersibles, often constrained by the complexity, power consumption, and bulk of conventional propulsion and control systems, stand to benefit enormously. The ability to switch drag profiles and generate lateral forces through surface texture modulation alone could yield submersibles that are not only more energy-efficient but also capable of accessing challenging environments with unparalleled precision. Applications may include environmental monitoring, infrastructure inspection, and covert surveillance in regions where traditional rigid hydrodynamic control surfaces are less effective or pose design challenges.
Looking forward, the research team envisions broadening this concept through synergistic collaborations, merging advanced materials science, soft robotics, and fluid mechanics. By integrating responsive polymers and actuators into the skin’s architecture, future prototypes could enable real-time resolution beyond the current vacuum-actuated dimples, opening avenues for even more refined manipulations of flow dynamics. This cross-disciplinary progress would push the frontier of “smart skins” for vehicles that can learn, adapt, and optimize their shapes continuously in response to ever-changing environmental conditions.
Lead author Anchal Sareen, assistant professor of naval architecture, marine engineering, and mechanical engineering at the University of Michigan, heralds this innovation as a potential game-changer. Traditional jointed control surfaces, such as ailerons, rudders, and fins, come at the cost of added weight, complexity, and susceptibility to mechanical failure. In contrast, a lightweight, morphable skin that modulates drag and lift properties could streamline vehicle architecture, reduce energy consumption, and enhance durability. This fluid, adaptive interface between vehicle and environment redefines interactive boundary conditions in fluid dynamics, fostering more robust and autonomous vehicle operations.
The research has already yielded two seminal peer-reviewed studies, published in the journals Flow and The Physics of Fluids, that detail the aerodynamic mechanisms and theoretical foundations underpinning the morphable skin’s drag and lift control capabilities. The experimental methodologies, combining precise force measurements with flow visualization, set a rigorous benchmark for future inquiry in this nascent field. Furthermore, graduate students and postdoctoral fellows involved have expressed enthusiasm about the surprisingly strong performance obtained with this relatively simple, elegant design, laying the groundwork for expanding this principle into diverse vehicular platforms.
In essence, the dynamic shape-changing skin represents a remarkable confluence of bioinspiration, material ingenuity, and classical fluid mechanics. It underscores how revisiting well-understood physical phenomena—such as the function of golf ball dimples—with fresh perspectives and modern technologies can unlock new technological paradigms. As unmanned systems proliferate in both the ocean’s depths and aerial domains, technologies like the Michigan morphable skin promise to redefine vehicle design metrics, enabling autonomous platforms to navigate complex environments more efficiently and responsively than ever before.
As we edge closer to a future where underwater and aerial vehicles seamlessly adapt their form to fluid conditions, the morphable dimpled skin technology paves an exciting path. By combining fundamental physics with cutting-edge engineering, this innovation not only illuminates new scientific understanding but also carries immense practical promise—emerging as a transformative solution for the next generation of agile, energy-conscious robotic explorers.
Subject of Research: Adaptive drag and lift control in underwater and aerial vehicles using morphable surface textures inspired by golf ball dimples.
Article Title: Dynamic Morphable Skins: Revolutionizing Vehicle Efficiency and Maneuverability by Harnessing Adaptive Dimpled Surfaces
News Publication Date: Not specified
Web References:
Adaptive drag reduction of a sphere using smart morphable skin (DOI: 10.1017/flo.2025.7)
On the lift generation over a sphere using asymmetric roughness (DOI: 10.1063/5.0241948)
References:
Sareen, A., Vilumbrales-Garcia, R., Sudarsana, P. B., et al., “Adaptive drag reduction of a sphere using smart morphable skin,” Flow, 2025.
Sareen, A., Vilumbrales-Garcia, R., Sudarsana, P. B., et al., “On the lift generation over a sphere using asymmetric roughness,” The Physics of Fluids, 2024.
Image Credits: Not provided
Keywords
Adaptive morphable skin, drag reduction, lift generation, fluid dynamics, underwater vehicles, aerial vehicles, smart surfaces, programmable textures, fluid–structure interaction, golf ball aerodynamics, naval architecture, soft robotics
Tags: aerodynamic efficiency in vehiclesAgile underwater vehiclesairborne robotics advancementsdimpled surface technologydrag and lift manipulationfluid dynamics in vehicle designgolf ball design inspirationinnovative vehicle maneuverabilitymorphable skin for vehiclesoceanic exploration technologypressure drag reduction techniquesUniversity of Michigan research breakthroughs
What's Your Reaction?
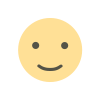
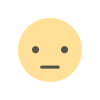
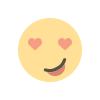
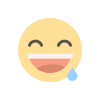
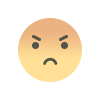
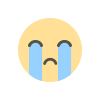
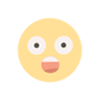