3D-Printed Scaffolds Transform Bone Repair
In recent years, the intersection of advanced manufacturing and regenerative medicine has given rise to groundbreaking strategies for repairing complex bone defects, a challenge that has long stymied even the most seasoned clinicians. Building on the transformative capabilities of 3D bioprinting, researchers are now capable of fabricating scaffolds that not only mimic the intricate architecture […]

In recent years, the intersection of advanced manufacturing and regenerative medicine has given rise to groundbreaking strategies for repairing complex bone defects, a challenge that has long stymied even the most seasoned clinicians. Building on the transformative capabilities of 3D bioprinting, researchers are now capable of fabricating scaffolds that not only mimic the intricate architecture of native bone but also integrate biomechanical and biological functionalities essential for successful regeneration. A newly published comprehensive review from Sun, Chen, Zhang, and colleagues in BioMedical Engineering OnLine meticulously delves into these innovations, shedding light on material advancements, structural biomechanics, and the future clinical prospects of 3D-printed bone scaffolds.
Large bone defects, often arising from trauma, tumor resection, or congenital malformations, present formidable obstacles in clinical settings. Traditional grafting techniques such as autografts and allografts carry significant limitations, including the scarcity of donor tissue and risks of immune rejection or infection. This underscores an urgent need for alternatives that can overcome these drawbacks while ensuring optimal integration and functionality. The reviewed work highlights how the precision of 3D printing technologies enables the design and fabrication of scaffolds tailored at multiple scales, from macrostructures supporting load-bearing to nano-level features enhancing cellular interactions.
Central to the review is the exploration of material innovation. The authors detail the synergy between metals, ceramics, polymers, and composite materials, engineered to replicate the mechanical stiffness and bioactivity of bone tissue. Metallic scaffolds, notably those based on titanium and its alloys, deliver superior mechanical strength required for structural support but suffer from bioinertness and potential stress shielding. To counter these weaknesses, composite approaches incorporate bioactive ceramics like hydroxyapatite and bioresorbable polymers, which enhance osteoconductivity and modulate degradation rates, harmonizing scaffold resorption with new bone formation.
Equally transformative is the structural design philosophy underscored throughout the review, where hierarchical porosity plays a pivotal role. The integration of macro, micro, and nano-porous architectures within scaffolds fosters vascularization, nutrient transport, and cellular infiltration—parameters essential for tissue integration and remodeling. Sophisticated computational modeling and biomechanical testing have paved the way for optimization strategies that calibrate pore size, shape, and interconnectivity to balance mechanical integrity with biological functionality. Such structural complexity is being realized through additive manufacturing techniques capable of multiscale precision.
Biological functionalization emerges as the third cornerstone of these scaffolds’ success. The review underscores advances in surface modification techniques and bioactive molecule delivery, where growth factors like BMP-2 are embedded for controlled release, stimulating bone regeneration pathways. Additionally, cell-seeding strategies employing mesenchymal stem cells or osteoprogenitor cells before implantation highlight efforts to precondition scaffolds toward osteogenic potential. Surface patterning and chemical modification further foster cell adhesion, proliferation, and differentiation, creating bioinstructive environments conducive to bone healing.
Despite these advances, the review does not shy away from discussing critical translational hurdles. The establishment of stable and functional vascular networks within large scaffolds remains a substantial bottleneck, limiting the viability of implanted constructs and their regenerative capacity. Strategies integrating angiogenic factor delivery and prevascularization techniques are intensively investigated but have yet to reach consistent clinical applicability. Moreover, the challenge of ensuring mechanical stability of scaffolds under physiological load-bearing conditions is intricately tied to both material selection and structural design, necessitating continued refinement.
Manufacturing scalability also represents a contentious frontier. While 3D bioprinting at lab scale demonstrates remarkable customization and precision, translating these methods into scalable, reproducible clinical-grade production demands improvements in reproducibility, standardization of bioinks, and regulatory alignment. The authors advocate for cross-disciplinary collaboration and the establishment of robust manufacturing pipelines to bridge this gap effectively, moving from proof-of-concept to widespread medical deployment.
Looking ahead, the review paints an exciting future shaped by novel concepts such as 4D dynamic scaffolds—structures that transform in response to environmental stimuli and adapt over time to the healing process. Stimuli-responsive smart biomaterials capable of modulating their properties—such as stiffness, degradation rate, or bioactive factor release—in reaction to biological cues, signify a leap toward truly intelligent implants. Moreover, the integration of artificial intelligence in the design phase holds promise for patient-specific optimization, harnessing data-driven algorithms to tailor scaffold architecture and composition to individual defect geometries and physiological requirements.
The convergence of these technological innovations opens new horizons for tackling the persistent clinical challenges in bone defect repair. The synergistic combination of advanced materials, hierarchical architecture, and biological cues within customized 3D-printed scaffolds positions this field at the vanguard of regenerative medicine. As the reviewed literature suggests, continued interdisciplinary research integrating materials science, biomechanics, biology, and computational modeling is paramount for translating these laboratory successes into transformative clinical therapies.
In sum, the review by Sun and colleagues provides a panoramic yet detailed overview of the cutting-edge advancements and imminent challenges poised to redefine the landscape of bone tissue engineering. Their analysis of scaffold materials, biomechanical considerations, biological functionalization, and clinical integration crystallizes the complexity and promise inherent in the field. These insights illuminate a path forward where biofabrication technologies not only restore bone but do so with unprecedented precision, intelligence, and efficacy.
The significance of this work resonates beyond academic circles, underscoring a paradigm shift that merges engineering innovation with clinical needs. As the global population ages and demands for effective musculoskeletal repair surge, the acceleration of scaffold technologies will likely catalyze new therapeutic paradigms. Ultimately, the fusion of 3D printing, biological science, and digital medicine lays a foundation for regenerative interventions that are as personalized as they are potent—a future where large bone defects become solvable challenges rather than intractable problems.
Subject of Research: Advances in 3D-printed scaffold technologies for bone defect repair, focusing on materials, biomechanics, and clinical applications.
Article Title: Advances in 3D-printed scaffold technologies for bone defect repair: materials, biomechanics, and clinical prospects.
Article References: Sun, J., Chen, C., Zhang, B. et al. Advances in 3D-printed scaffold technologies for bone defect repair: materials, biomechanics, and clinical prospects. BioMed Eng OnLine 24, 51 (2025). https://doi.org/10.1186/s12938-025-01381-w
Image Credits: AI Generated
DOI: https://doi.org/10.1186/s12938-025-01381-w
Tags: 3D-printed bone scaffoldsadvanced manufacturing in healthcareautografts and allografts limitationsbiomechanical properties of scaffoldsbone defect repair technologieschallenges in bone grafting techniquesclinical applications of 3D printingfuture prospects of bioprintingintricate architecture of native bone.material advancements in bioprintingregenerative medicine innovationstailored scaffold design for bone regeneration
What's Your Reaction?
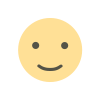
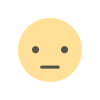
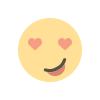
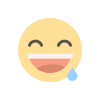
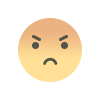
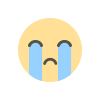
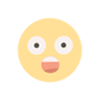