Transforming Robot Collectives: Creating Smart Material Behavior in Robotics
Researchers at UC Santa Barbara and TU Dresden are pioneering a groundbreaking advancement in the field of robotics, creating a collective of robots that behaves much like a material. This innovative concept strives to bridge the gap between traditional robotics and material science, where robotics can mimic the remarkable characteristics of biological materials. The lead […]

Researchers at UC Santa Barbara and TU Dresden are pioneering a groundbreaking advancement in the field of robotics, creating a collective of robots that behaves much like a material. This innovative concept strives to bridge the gap between traditional robotics and material science, where robotics can mimic the remarkable characteristics of biological materials. The lead researcher, Matthew Devlin, presents a clear vision for the future of robotic systems that can adapt, transform, and even exhibit properties similar to those found in living organisms.
The foundational work centers on a collection of disk-shaped autonomous robots, designed to resemble small hockey pucks. These robots have been programmed to assemble into diverse shapes and configurations, effectively behaving like a new type of material with distinct properties. The researchers have ventured into the complex realm of material properties, where the robots demonstrate the ability to be both strong and rigid, yet fluid and adaptable, depending on the task or form required.
A remarkable aspect of this robotic system is how it draws inspiration from living systems, particularly from embryonic tissues. Researcher Otger Campàs, who previously worked at UCSB and is now at TU Dresden, delineates that living tissues possess extraordinary capabilities such as self-healing, self-shaping, and manipulating their material strength in response to different stimuli. This biological insight drives the design in which robots can flexibly switch between solid and fluid states, akin to how cells in an embryo reorganize as they develop.
The collaboration between mechanical engineering and biological studies yields insight into the mechanics behind these processes. Emergent properties of the robots are controlled not only by external forces but also by intricate internal mechanisms. This internal signaling allows the robots to coordinate their movements and adjust their shapes dynamically—an ability that could revolutionize fields like robotics and material science. During the developmental processes of embryos, cells interact through numerous active forces, leading to a profound reorganization from a formless collection into structured forms like limbs and organs. Similarly, by imitating these forces in their robotic systems, researchers can generate transformative capabilities for the robots.
Illustrating the theoretical underpinnings, scientists implemented mechanical components in the robots that facilitate this inter-unit force. This is achieved through eight motorized gears located on the circumference of each robot, enabling them to navigate around one another and constructively push each other even in confined spaces. Such mechanical manipulation mirrors the natural interactions observed in living organisms, where cells work harmoniously to reshape the material they compose.
Moreover, the researchers have utilized light sensors equipped with polarized filters that serve as a method for the robots to perceive their environment. When exposed to targeted light, these sensors direct the robots to rotate their gears in specific ways, altering their formation effortlessly. This feature allows for synchronized movements within the collective, as robots can respond collectively to changes in light, much like how cells respond to biochemical signals in embryonic development.
A particularly fascinating area of study within this research involves the concept of adhesion among the robots. This adhesion is achieved through strategically integrated magnets on the edges of the robotic units. Activation or deactivation of these magnets allows robots to attract or repel each other as necessary, cultivating a more cohesive or fluid collective when interacting with various environments or tasks.
Research findings demonstrate that fluctuations in signal strength are pivotal to enabling shape-shifting capabilities in this robotic ensemble. By simulating the natural unpredictability found in cell interactions, researchers noted improved fluidity and adaptability in the robotic collective. A key takeaway is that robots mimic biological systems in converting solid states to fluid states based on these fluctuations, enhancing their ability to respond to environmental changes.
By modulating both signal fluctuations and inter-unit forces, the researchers successfully transformed the collective from a rigid configuration to a dynamic, flowing entity. This approach not only reduces overall power consumption but unlocks potential for advanced robotic applications. Dynamic switching between these states means that robots can perform tasks efficiently by conserving energy when rigid and activating movement when flow is required.
These developments suggest broader applications beyond mere robotics—they pave the way for further investigations into the principles of active matter and phase transitions. The findings could offer fresh insights into biological research, opening pathways to understanding how living systems function at a material level. This powerful interplay between robotics and biology could potentially lead to unprecedented advances in intelligent materials and adaptive systems.
As scientific exploration continues, the potential of scaling down these robotic units leads towards the creation of larger, more versatile assemblies that can function much like traditional materials but with inherent active capabilities. Researchers are optimistic about leveraging machine learning strategies to unlock new behaviors and enhance the usability of such robotic systems. The work done by Devlin, Hawkes, and their team signifies an exciting step forward in redefining what materials can do, encouraging a wave of innovation that merges material science with robotics.
With this foundational knowledge and continuing exploration, the researchers envision expansive applications for their material-like robotic collectives. By integrating sophisticated controls and self-regulatory mechanisms, they aim to develop robotic systems that can evolve beyond their initial programming. The possibilities are endless as they explore how these robots can reshape themselves, handle substantial loads, or even demonstrate self-healing abilities, much like biological materials.
As the world increasingly turns towards automation and intelligent systems, this groundbreaking research embodies a critical intersection between technology and biology, epitomizing the future of materials science. The implications stretch beyond academic curiosity, as we contemplate a new era of smart materials that can adapt to the environment, self-arrange, and ultimately enhance human capabilities in multifaceted and unforeseen ways.
Subject of Research: Material-like robotic collectives with spatiotemporal control of strength and shape
Article Title: Material-like robotic collectives with spatiotemporal control of strength and shape
News Publication Date: 21-Feb-2025
Web References: Science
References: 10.1126/science.ads7942
Image Credits: N/A
Keywords
Applied sciences and engineering
Robotics
Mechatronics
Robot control
Robotic designs
Swarm robotics
Robotic locomotion
Tags: adaptive robotic systemsautonomous robot designbio-inspired roboticsbridging robotics and biologycollaborative robots in engineeringdisk-shaped autonomous robotsemergent behavior in roboticsmaterial science in roboticsrobotic collectivesself-healing robotic systemssmart material behavior in roboticstransforming robotic structures
What's Your Reaction?
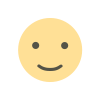
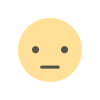
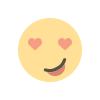
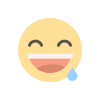
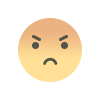
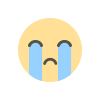
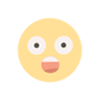