Texas A&M chemists, geologists bond over NSF-funded study of clumped isotopes
Carbon dioxide levels in Earth’s atmosphere — and, consequently, ocean temperatures — are rising. How high and how fast ocean temperatures can rise can be learned from temperature measurements of ancient oceans. At the same time, energy exploration also relies on knowing the thermal history of oil and gas source rocks, which is often difficult […]

Carbon dioxide levels in Earth’s atmosphere — and, consequently, ocean temperatures — are rising. How high and how fast ocean temperatures can rise can be learned from temperature measurements of ancient oceans. At the same time, energy exploration also relies on knowing the thermal history of oil and gas source rocks, which is often difficult to determine.
Credit: Courtesy of Dr. Ethan Grossman
Carbon dioxide levels in Earth’s atmosphere — and, consequently, ocean temperatures — are rising. How high and how fast ocean temperatures can rise can be learned from temperature measurements of ancient oceans. At the same time, energy exploration also relies on knowing the thermal history of oil and gas source rocks, which is often difficult to determine.
One of the most promising techniques for measuring ancient ocean temperatures and basin thermal histories relies on the co-enrichment of rare heavy oxygen and heavy carbon in the calcium carbonate compound found at the bottom of the ocean. This enrichment, termed clumped isotopes, is commonly measured using fossil shells and limestones to determine the temperatures at the time when sediments became deposited on the sea floor.
However, there’s a catch: Clumped isotope temperatures can be reset by the very process of sediments being buried, causing those sediment temperatures to rise as they create the same conditions responsible for converting organic matter in sedimentary rocks to oil.
Such complex problems require interdisciplinary approaches — a collaborative mindset that thrives in the Texas A&M University College of Arts and Sciences, where a team of geologists and chemists has taken the quest to the atomic level to more accurately measure ancient ocean temperatures.
The team, led by Dr. Ethan Grossman in the Department of Geology and Geophysics and Dr. Sarbajit Banerjee in the Department of Chemistry, recently used a combination of supercomputing and density functional theory to model the process responsible for setting and resetting clumped isotope compositions, a phenomenon known as reordering.
“We were able to vividly simulate the motion of atoms and to capture the entire process underpinning rearrangement of carbon-oxygen bonds,” said Grossman, holder of the Michel T. Halbouty Chair and co-director of the Stable Isotope Geosciences Facility at Texas A&M. “This modeling technique, commonly applied to simulate the behavior of atoms in many scenarios, including lithium-ion batteries and brain-like computing, is for the first time being used to examine the rare movement of atoms in fossil shells and limestone rock.”
In comparing their results to previously published experimental results, Grossman says the team was also able to provide the missing link between experimentation and theory in identifying the catalytic culprit responsible for speeding up temperature resets in those clumped isotopes: water.
“We theoretically demonstrated for the first time that water in the crystal structure will hasten the resetting of clumped isotope temperatures, which thus warrants caution for how the approach is used to reconstruct ancient temperature records,” Grossman added. “This supports experimental data that previously lacked a theoretical underpinning and will lead to more accurate reconstructions of past climates, which in turn provides understanding of future climate scenarios.”
In addition to identifying the role of water as an accelerant in reordering, Grossman says the team’s studies help explain other enigmatic results — notably, the modification of fossil-derived ocean temperatures to impossibly high values hovering around 150 degrees Celsius, or roughly 300 degrees Fahrenheit. They were able to determine such outliers using specimens from approximately 320-million-year-old marine sedimentary rock deeply buried in the past and now exposed in New Mexico and the Ural Mountains in Russia.
“Clearly, these organisms did not live in water hotter than boiling temperatures,” he explained. “This finding pointed to the need to understand the burial history of fossils and the rates of clumped isotope reordering.”
The team’s results, published earlier this summer in Science Advances, represent a pivotal first step in developing a unified theory for the clumped-isotope reordering kinetics in carbonate minerals that Grossman says will pave the way for more accurate determinations of ancient ocean temperatures and the thermal history of petroleum basins. By illustrating how activation energy barriers and reordering rates are modified by crystal defects, ion substitution and incorporated water, they hope to contribute to more accurate reconstructions of past climates and a clearer understanding of future climate scenarios while also providing a mechanism for reconstructing the thermal history of sedimentary basins essential for oil and gas exploration.
“This study will allow for more accurate reconstructions of past climates by better understanding the depths of sediment burial beyond which clumped isotope temperatures from fossil shells are unreliable,” Grossman said. “Furthermore, it demonstrates the value of collaboration between departments and fields that have not traditionally worked together to unveil fundamentally new knowledge.”
In addition to Grossman and Banerjee, the team’s paper featured three lead co-authors: 2021 Texas A&M chemical engineering Ph.D. graduate Dr. Saul Perez-Beltran ’20, who currently is a postdoctoral researcher with the Texas A&M Engineering Experiment Station; 2023 Texas A&M materials and analytical chemistry Ph.D. graduate Dr. Wasif Zaheer ’19, now a senior research specialist with Dow; and current Texas A&M geology candidate Zeyang Sun ’19. The University of Queensland’s Dr. William F. Defliese, formerly a Berg-Hughes Postdoctoral Fellow at Texas A&M from 2017-2019, also was involved in the work.
The team’s paper, “Density Functional Theory and Ab-Initio Molecular Dynamics Reveal Atomistic Mechanisms for Carbonate Clumped Isotope Reordering,” can be viewed online along with related figures and acknowledgements. Their research was funded by the National Science Foundation (Grant No. EAR-19115647), with additional support from The Welch Foundation (Grant No. A-A1978-20220331), the Michel T. Halbouty Chair in Geology and the Chancellor’s Research Initiative for Mass Spectrometry, established in 2016.
“We have many unanswered questions,” Grossman said. “For starters, how does this reordering rate vary with the amount of water in the crystal structure? How does it vary in different minerals? Can we develop protocols for identifying the fossil and mineral material most resistant to reordering? Can we define a calibration scale to correct for errors in each mineral? And lastly, can we use this information to develop a new and innovative approach to reconstruct basin thermal histories and refine oil and gas exploration? In sum, we are eager for our next steps.”
Journal
Science Advances
DOI
10.1126/sciadv.adf170
Article Title
Density functional theory and ab initio molecular dynamics reveal atomistic mechanisms for carbonate clumped isotope reordering
Article Publication Date
28-Jun-2023
What's Your Reaction?
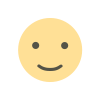
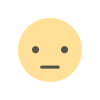
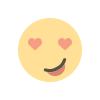
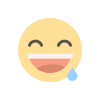
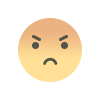
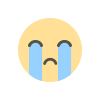
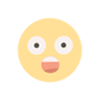