Tabletop High-Energy Proton Accelerator Powered by University-Class Lasers
In a groundbreaking advancement that promises to revolutionize laser-driven ion acceleration, researchers at the Tata Institute of Fundamental Research (TIFR) Hyderabad have developed a method to generate high-energy protons using comparatively low-energy laser pulses operating at unprecedented repetition rates. This innovative approach challenges conventional beliefs, which have long held that only massive, multi-joule laser systems […]

In a groundbreaking advancement that promises to revolutionize laser-driven ion acceleration, researchers at the Tata Institute of Fundamental Research (TIFR) Hyderabad have developed a method to generate high-energy protons using comparatively low-energy laser pulses operating at unprecedented repetition rates. This innovative approach challenges conventional beliefs, which have long held that only massive, multi-joule laser systems can produce ions accelerated to energies in the million electronvolt (MeV) range. By cleverly leveraging laser pre-pulses—historically regarded as detrimental artifacts—the TIFR team has opened a new frontier for compact, high-throughput ion accelerators suitable for broader scientific and technological applications.
Laser ion acceleration research has experienced burgeoning interest due to its potential applications spanning from medical therapies to advanced imaging and nuclear fusion. Conventionally, these acceleration mechanisms require intense laser pulses that heat a solid target’s electrons to extreme temperatures, effectively creating a plume of highly energetic ions. To emulate the electrostatic potentials of conventional particle accelerators—often spanning millions of volts—huge laser infrastructures delivering several joules per pulse are employed. However, these large-scale systems suffer from low repetition rates, typically only a few pulses per second, limiting their practical deployment outside of specialized research facilities.
An enduring challenge has been the trade-off between laser pulse energy and repetition rate. Smaller lasers, capable of firing thousands of times per second, deliver pulse energies measured in milli- or microjoules and have long been judged insufficient to achieve ion energies crossing into the MeV range. Established laser-ion acceleration phenomena at such low energies predict maximum ion energies in the kiloelectronvolt (keV) range, rendering high-energy proton production seemingly unfeasible. It is within this context that the TIFR group’s achievement represents a significant disruption to established paradigms, merging high particle energies with rapid repetition at minimal laser input energy.
Central to their technique is the reimagining of pre-pulses—low-intensity laser bursts occurring prior to a main intense pulse. Normally considered undesirable, pre-pulses tend to degrade the target surface before the primary pulse arrives, thereby diminishing the efficiency of ion acceleration and necessitating complex equipment to suppress them. Rather than eliminating pre-pulses, the researchers ingeniously utilize them to sculpt the target material, forming a hollow cavity within a micrometer-sized liquid droplet of methanol. This cavity transitions into a low-density plasma environment when irradiated, fundamentally altering the interaction dynamics of the subsequent intense laser pulse.
Once the main laser pulse enters this plasma cavity, it triggers a pair of colossal plasma waves through a phenomenon known as the two-plasmon decay instability. These counterpropagating waves grow to immense amplitude but rapidly collapse as they move through the plasma, releasing bursts of highly energetic electrons. These electrons, in turn, create robust localized electric fields capable of accelerating protons to energies reaching hundreds of kiloelectronvolts and beyond, surmounting the limits traditionally imposed by low-energy laser drivers.
What sets this approach apart is not merely the acceleration of ions to MeV-scale energies, but the high repetition rate of operation—up to a thousand pulses per second using few-millijoule laser pulses. This high-throughput capability is crucial for real-world applications, such as targeted cancer therapy where dose delivery and control over ion beams over numerous pulses are essential. Equally important is the method’s scalability and relative simplicity compared to existing techniques that rely on synchronization and suppression of parasitic pre-pulses. By converting a longstanding complication into an operational advantage, this method opens the door for university labs worldwide to explore laser ion acceleration without recourse to massive laser installations.
The implications of producing MeV protons using modest millijoule lasers extend far beyond academic curiosity. Ion beams generated in this way show considerable promise in non-destructive evaluation of materials, particle radiography, and even inertial confinement fusion research where precise control over plasma conditions is vital. The ability to produce high-energy ions at kilohertz repetition rates means data collection can be significantly accelerated, facilitating real-time monitoring and iterative experimental protocols, a stark contrast with the ponderous timescales of existing large laser facilities.
Technically, the method hinges upon careful synchronization and tuning of the laser pre-pulse properties as they interact with the liquid target. The pre-pulse effectively “prepares” the target by carving out the plasma cavity, defining the initial conditions for the two-plasmon decay process. This intricate interplay between laser timing, plasma density, and cavity geometry determines the efficiency and energy of the accelerated ions. Such detailed plasma engineering—once largely impractical—becomes central to the process, offering diverse knobs to optimize performance.
Beyond the experimental setup, the TIFR team’s work further deepens our theoretical understanding of laser-plasma instabilities and their role in ion acceleration. The two-plasmon decay instability, often considered a parasitic effect that siphons energy away from intended processes, is here harnessed to amplify electron production. The resulting electron bursts create intense sheath fields, which are the actual accelerators for the protons. This nuanced perspective underscores the importance of embracing complex plasma dynamics instead of attempting to suppress them outright.
The reproducibility and stability of the ion beams obtained are also noteworthy; the use of liquid microdroplet targets ensures a self-refreshing surface, preventing degradation issues common to solid targets bombarded at high repetition rates. This makes the system far more sustainable and suitable for continuous operation, an essential attribute for applications demanding extended runtime.
Furthermore, the liquid target aspect introduces flexibility in target composition and geometry, potentially allowing tailoring of ion species and beam characteristics. By modifying the liquid medium or adjusting droplet size, researchers can fine-tune acceleration parameters to meet specialized requirements. This adaptability enhances the versatility of laser-driven ion acceleration systems derived from this approach.
Collectively, the TIFR Hyderabad study signals a paradigm shift, challenging the dogma that only large, complex laser facilities can produce high-energy ion beams. Through elegant exploitation of pre-pulse effects and liquid target dynamics, the researchers have demonstrated a practical pathway to scalable, tabletop ion accelerators operating at rates and energies previously thought unattainable in small-scale systems. This opens myriad opportunities for widespread adoption in medical physics, materials science, and fundamental plasma research.
This breakthrough also highlights the broader trend of re-examining perceived limitations in laser-matter interaction and plasma physics as opportunities. By turning the once-problematic laser pre-pulse into a facilitator of plasma wave generation and ion acceleration, the study exemplifies how innovative approaches can overturn long-standing technical roadblocks, accelerating progress toward compact, high-efficiency particle accelerators accessible to a larger scientific community.
The full details of this research, including experimental methodology, results, and theoretical analyses, have been published in the journal Physical Review Research under the title “High-repetition rate ion acceleration driven by a two-plasmon decay instability.” This publication not only disseminates these findings but provides a valuable resource for researchers aiming to build upon this promising approach, thereby advancing the frontiers of laser-driven ion acceleration technology.
Subject of Research: Ion acceleration using laser-driven plasma instabilities with high repetition rates and low laser pulse energies.
Article Title: High-repetition rate ion acceleration driven by a two-plasmon decay instability
News Publication Date: 4-Mar-2025
Web References:
https://journals.aps.org/prresearch/abstract/10.1103/PhysRevResearch.7.013240
http://dx.doi.org/10.1103/PhysRevResearch.7.013240
References:
S.V. Rahul, R. Sabui et al., Phys. Rev. Research 7, 013240 (2025)
Image Credits:
The image has been created by the authors
Keywords
Laser ion acceleration, plasma waves, two-plasmon decay instability, high repetition rate lasers, proton acceleration, low-energy laser pulses, vacuum plasma interactions, liquid microdroplet targets, plasma instabilities, medical applications of ion beams, compact accelerators, laser pre-pulse accommodation
Tags: advanced imaging techniquescompact ion acceleratorshigh-energy protons generationhigh-throughput acceleration methodslaser pre-pulses innovationlaser-driven ion accelerationlow-energy laser pulsesmedical therapies applicationsnuclear fusion technologyparticle accelerator alternativestabletop proton acceleratorTIFR Hyderabad research
What's Your Reaction?
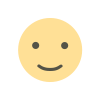
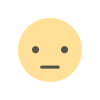
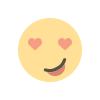
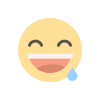
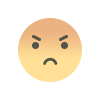
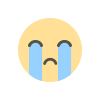
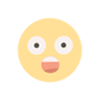