Superconductivity and Spin Canting in Trilayer Graphene
In recent years, the exploration of flat-band systems within two-dimensional materials has dramatically transformed our understanding of correlated electronic phases. Among these, graphene and transition metal dichalcogenides (TMDs) have emerged as a particularly compelling platform, showcasing a rich interplay of magnetism and superconductivity. These materials, when carefully engineered to form moiré superlattices or twisted layers, […]

In recent years, the exploration of flat-band systems within two-dimensional materials has dramatically transformed our understanding of correlated electronic phases. Among these, graphene and transition metal dichalcogenides (TMDs) have emerged as a particularly compelling platform, showcasing a rich interplay of magnetism and superconductivity. These materials, when carefully engineered to form moiré superlattices or twisted layers, manifest remarkable phase diagrams that reveal unconventional magnetic orders alongside elusive superconducting states. As the scientific community delves deeper into these phenomena, a key question has persisted: does magnetic ordering impede superconductivity, or can it foster the pairing mechanisms vital to robust superconducting phases?
This question has gained renewed urgency in the context of Bernal bilayer graphene, a bilayer form of graphene with specific stacking arrangements, which exhibits enhanced superconductivity under the influence of spin–orbit coupling introduced via proximity effects. Recent experiments show significant amplifications of superconducting domain sizes and critical temperatures, denoted as T_c, when spin–orbit interactions are strengthened. This association hints that spin–orbit coupling might play a pivotal role in stabilizing or even promoting superconductivity, though the precise mechanisms have remained elusive.
Now, a groundbreaking study takes this inquiry further by investigating rhombohedral trilayer graphene (RTG), a material characterized by three graphene layers stacked in a specific rhombohedral sequence. By introducing spin–orbit coupling through a sophisticated substrate proximity effect, researchers have discovered new superconducting “pockets” on both electron- and hole-doped sides of the phase diagram. Remarkably, the maximum critical temperature in these pockets reaches approximately 300 millikelvin—a threefold increase compared to RTG devices encapsulated solely by hexagonal boron nitride, the traditional insulating substrate typically used in these experiments.
The experimental approach employs sensitive local magnetometry, allowing for unprecedented insight into the magnetic landscape underpinning the superconducting phases. It reveals that superconductivity emerges precisely at a delicate transition between two distinct magnetic states. On one side lies a spin-canted state characterized by a finite in-plane magnetic moment, where electron spins are neither fully aligned nor anti-aligned but instead form an angle—a canting. On the other side resides a state defined by complete spin–valley locking, wherein spin and valley degrees of freedom become intertwined, restricting the system’s magnetic configuration. This coexistence and competition between spin textures mark a critical point intimately linked to the superconducting behavior.
Complementing these experimental findings, Hartree–Fock theoretical calculations provide a robust framework to understand the underlying physics. They show that the phase transition observed experimentally is a natural consequence of competition between spin–orbit coupling strength and the Hund’s interaction—a carrier-density-dependent exchange interaction that tends to align spins within localized orbitals. Crucially, tuning carrier density manipulates this competition, shifting the balance between the canted magnetic state and the spin-locked state. The theoretical model thus offers a comprehensive narrative connecting spin textures, coupling mechanisms, and electronic correlations to the emergence of superconductivity.
What makes this discovery particularly compelling is that the enhancement of superconductivity does not arise from a radical change in the fundamental symmetry or character of the ground state. Instead, it is tied to a more subtle, quantitative modification of the canting angle within the spin texture. This insight challenges previous assumptions that new or exotic phases of matter were necessary to explain improved superconducting properties. Instead, it points toward the nuanced role of magnetic fluctuations associated with the canting angle, which may actively contribute to the pairing interaction responsible for superconductivity.
This conceptual breakthrough aligns with recently proposed theoretical frameworks suggesting that fluctuations in spin-canting order parameters can act as a glue facilitating electron pairing. In spin–orbit-coupled environments, these magnetic dynamics become intertwined with the extremely sensitive electronic structure of trilayer graphene, enhancing superconducting correlations. Such a mechanism suggests a new paradigm where spin–orbit engineering offers a tunable handle on both magnetic order and superconducting pairing symmetry, opening avenues for designing quantum materials with tailored properties.
Furthermore, the findings highlight the importance of substrate engineering in van der Waals heterostructures. The proximity-induced spin–orbit coupling, leveraged here via the choice of substrate, emerges as a key experimental lever, enabling researchers to finely tune the interplay between spin, valley, and charge degrees of freedom. This finely orchestrated control surpasses previous methods that relied solely on electrostatic gating or twist angle variation, marking a new frontier in material design for quantum technologies.
The experimental techniques employed are equally notable. By combining high-sensitivity local magnetometry with transport measurements, the researchers offer a comprehensive picture that correlates microscopic magnetic phenomena with macroscopic superconducting properties. This dual approach not only enhances the reliability of the data but also provides a blueprint for future studies aiming to disentangle complex correlated states in two-dimensional materials.
It is worth emphasizing that these results do not only enrich our fundamental understanding of two-dimensional superconductivity but also bear practical significance. Elevating the critical temperature and improving the robustness of superconducting states in graphene-based systems could pave the way for ultra-low-power electronic devices and quantum information platforms. The ability to harness spin–orbit coupling for these ends adds a layer of versatility that could drive innovations in spintronics and topological quantum computing.
Moreover, the delicate balance uncovered between spin-canted magnetism and superconductivity echoes broader themes in condensed matter physics, where intertwined orders often dictate the emergent behavior of correlated electrons. This study thus adds a crucial piece to the puzzle of how intricate magnetic interactions can coexist with, or even bolster, unconventional superconducting states—an area that has long captivated physicists due to its profound theoretical challenges and technological potentials.
Looking ahead, numerous questions arise from this work. How universal is this spin–orbit-enhanced superconductivity across other multilayer graphene assemblies or transition metal dichalcogenide moiré systems? Can the canting angle and associated fluctuations be externally manipulated in real time to switch superconducting states on or off? And to what extent can theories be extended to predict other exotic phases stabilized by the combined effects of spin–orbit coupling, electronic correlations, and lattice symmetries?
In sum, the revelation that spin–orbit coupling can dramatically enhance superconductivity by tuning spin canting in rhombohedral trilayer graphene represents a major advance in the burgeoning field of two-dimensional quantum materials. This study not only provides fresh experimental evidence but also deepens theoretical insight into the subtle interplay between competing orders that define emergent quantum phases. As the search for novel superconductors intensifies, spin–orbit engineering in layered graphene systems stands poised to become a central strategy for realizing and controlling next-generation quantum states.
Subject of Research: Spin–orbit coupling effects and superconductivity in rhombohedral trilayer graphene.
Article Title: Superconductivity and spin canting in spin–orbit-coupled trilayer graphene.
Article References:
Patterson, C.L., Sheekey, O.I., Arp, T.B. et al. Superconductivity and spin canting in spin–orbit-coupled trilayer graphene. Nature (2025). https://doi.org/10.1038/s41586-025-08863-w
Image Credits: AI Generated
Tags: Bernal bilayer graphene propertiescorrelated electronic phases in 2D materialscritical temperatures in superconductorsengineering flat-band systems in materialsmagnetic ordering and superconducting phasesmoiré superlattices and superconductivityrhombohedral trilayer graphene studyspin canting in trilayer graphenespin-orbit coupling effects in graphenesuperconductivity in graphenetwo-dimensional materials and magnetismunconventional magnetic orders in graphene
What's Your Reaction?
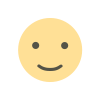
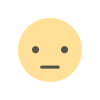
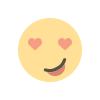
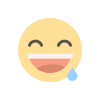
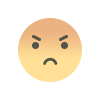
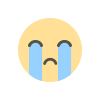
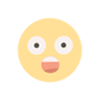