Subnanosecond Flash Memory via 2D Injection
In a groundbreaking development poised to revolutionize the field of non-volatile memory technologies, researchers have harnessed the unique properties of two-dimensional (2D) materials to shatter long-standing speed barriers of flash memory devices. By innovatively exploiting the atomic-scale thickness of 2D structures, the team demonstrated a novel mechanism termed 2D-enhanced hot-carrier injection (2D-HCI), effectively enabling program […]

In a groundbreaking development poised to revolutionize the field of non-volatile memory technologies, researchers have harnessed the unique properties of two-dimensional (2D) materials to shatter long-standing speed barriers of flash memory devices. By innovatively exploiting the atomic-scale thickness of 2D structures, the team demonstrated a novel mechanism termed 2D-enhanced hot-carrier injection (2D-HCI), effectively enabling program speeds on the order of hundreds of picoseconds. This breakthrough marks the first time that flash memory devices have reliably operated below the one-nanosecond programming speed threshold, charting a new course for ultra-fast data storage technologies.
The crux of this advancement lies in the intrinsic electric field distribution within ultra-thin channels composed of 2D materials such as graphene and other atomically thin semiconductors. Traditional flash memory devices have typically been hindered by limitations in carrier acceleration efficiency, primarily due to relatively thick silicon channels and inefficient electric field modulation. However, in 2D materials, the channel thickness is reduced to the atomic scale, drastically altering the distribution of the transverse electric field component, denoted as Ey, across the channel.
This confinement of the electric field facilitates a channel-thickness-modulated Ey distribution, which markedly improves the acceleration of carriers within the channel. The enhanced electric field effectively injects carriers into the floating gate or charge trapping layer with unprecedented speed and efficiency — a process fundamental to the programming cycle of flash memory. The result is a robust mechanism that accelerates the hot-carrier injection process, thus enabling program speeds unattainable by traditional three-dimensional semiconductor architectures.
To validate the 2D-HCI concept, the research team fabricated flash memory devices integrating ultra-thin graphene channels. These experimental devices demonstrated a program time of just 400 picoseconds, an order of magnitude faster than currently deployed non-volatile flash memories, which generally operate near or above the nanosecond regime. This performance leap is not only significant for its speed but also for its endurance and reliability, as the 2D-HCI devices showed remarkable stability across repeated programming cycles.
One of the standout features of the 2D-HCI mechanism is its compatibility with a broad spectrum of 2D materials, including both Dirac materials like graphene and 2D semiconductors such as transition metal dichalcogenides. This universality suggests that the approach is not limited to a single material system but can be optimized and adapted across varying atomic-scale platforms, further broadening the potential impact.
This discovery challenges the pre-existing constraints faced by scaling laws in semiconductor device engineering. Traditionally, reducing channel length and thickness has been a double-edged sword due to short-channel effects and increased leakage currents. Yet, the use of atomically thin materials circumvents many of these issues by providing inherent electrostatic control and reducing parasitic capacitances, paving the way for aggressive device miniaturization while simultaneously improving performance metrics.
Beyond sheer speed improvements, the 2D-HCI mechanism also promises enhancements in power efficiency. The improved carrier acceleration reduces the voltage and energy required for programming operations, a critical consideration for mobile and edge computing applications where energy budgets are stringent. With data centers increasingly seeking low-latency, energy-efficient storage solutions, such innovations could provide significant competitive advantages.
The potential to scale the device performance further by shortening the channel length opens exciting avenues for next-generation memory design. As device dimensions trend towards the nanoscale, combining 2D channel materials with advanced lithographic techniques could yield ultra-compact, high-speed memory cells primed for integration into silicon-based platforms or even flexible electronics.
From a fabrication perspective, integrating 2D materials into conventional semiconductor manufacturing remains a challenge, yet recent advancements in wafer-scale synthesis and transfer techniques have greatly improved the viability of these materials for industrial applications. The successful demonstration of reliable 2D-HCI devices underscores the maturity of these processes and the practical feasibility of commercial deployment in the near future.
Moreover, the findings suggest that the 2D-HCI mechanism might transcend memory applications alone. The efficient and rapid injection of hot carriers enabled by atomically thin channels could inspire innovations in other device architectures requiring fast charge transfer processes, such as sensors, logic devices, and neuromorphic computing elements.
Fundamentally, this work unlocks new understandings in hot-carrier dynamics at the atomic scale. By carefully modulating the electric field distribution through engineering of channel thickness, the study reveals how quantum-confined systems can drastically alter carrier behavior, providing a fresh perspective for device physicists and materials scientists alike.
The implications of enabling sub-nanosecond programming speeds extend deeply into the future of computing, where demands for rapid data access and high-throughput storage continue to escalate. With ever-intensifying workloads driven by artificial intelligence, big data analytics, and augmented reality, the necessity for fast, reliable, and energy-efficient non-volatile memory technologies has never been more pressing.
In summary, the successful realization of subnanosecond flash memory programming via 2D-enhanced hot-carrier injection introduces a paradigm shift with transformative potential. By leveraging the extraordinary properties of 2D materials, this research bridges fundamental physics and practical device engineering, heralding a new era in ultra-fast, robust, and scalable memory devices that could reshape the landscape of digital storage technologies worldwide.
Subject of Research: Subnanosecond programming of flash memory enabled by two-dimensional material-induced hot-carrier injection mechanisms.
Article Title: Subnanosecond flash memory enabled by 2D-enhanced hot-carrier injection.
Article References:
Xiang, Y., Wang, C., Liu, C. et al. Subnanosecond flash memory enabled by 2D-enhanced hot-carrier injection. Nature (2025). https://doi.org/10.1038/s41586-025-08839-w
Image Credits: AI Generated
Tags: 2D-enhanced hot-carrier injectionatomic-scale thickness in semiconductorsbreakthroughs in data storage devicescarrier acceleration efficiency improvementselectric field distribution in memory devicesgraphene in memory technologyinnovative semiconductor technologiesnon-volatile memory advancementsprogramming speed in flash memorysubnanosecond flash memorytwo-dimensional materials in electronicsultra-fast data storage technologies
What's Your Reaction?
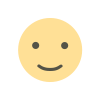
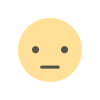
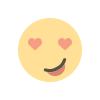
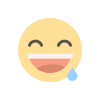
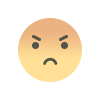
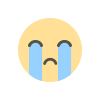
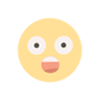