Speeding Up Bacterial Evolution in the Lab: New Advances Unveiled
A groundbreaking advance in experimental evolutionary biology has emerged from the laboratories of the University of Tokyo, where researchers have developed a pioneering system capable of dramatically accelerating the evolution of bacterial genome structures. This breakthrough hinges on exploiting the power of “jumping genes,” specifically DNA elements known as insertion sequences (ISs), to induce rapid, […]

A groundbreaking advance in experimental evolutionary biology has emerged from the laboratories of the University of Tokyo, where researchers have developed a pioneering system capable of dramatically accelerating the evolution of bacterial genome structures. This breakthrough hinges on exploiting the power of “jumping genes,” specifically DNA elements known as insertion sequences (ISs), to induce rapid, large-scale genomic rearrangements in Escherichia coli (E. coli). This methodology not only opens unprecedented avenues for direct observation of evolutionary processes but also challenges longstanding paradigms about genome dynamics and complexity.
The architecture of an organism’s genome—how genes are arranged and organized within DNA—serves as a fundamental determinant for cellular functions, organismal biology, and evolutionary trajectories. Yet, studying the natural evolution of genome structure in real-time has remained elusive because it often unfolds over scales of decades or centuries. Traditional laboratory evolution experiments typically monitor small-scale genetic mutations but fail to capture the broader genome-wide structural changes. The team, led by Yuki Kanai and colleagues, has now bridged this gap by harnessing the evolutionary potential of active insertion sequences to simulate decades of genomic remodeling within mere weeks.
Insertion sequences are mobile genetic elements capable of “jumping” or transposing their position within a bacterial chromosome. These transpositions can result in mutations, duplications, deletions, or other reconfigurations that profoundly impact genome structure and function. However, under natural and standard laboratory conditions, the frequency of such IS transpositions in E. coli is exceedingly low, occurring approximately once per year or every few thousand generations. This slow pace has historically hampered detailed studies on their evolutionary influence. The innovation from the University of Tokyo team lies in their ability to introduce multiple copies of hyperactive IS elements into the bacterial genome, thus simulating a hypermutagenic environment that sharply elevates transposition events.
The inspiration for this approach was serendipitous, emerging from interdisciplinary collaboration. Studies on insect-associated bacteria revealed genomes that are dramatically reduced in size—around one-tenth the size of related free-living bacteria. These shrunken genomes were enriched in transposons, mobile elements thought to accelerate genome reduction by facilitating DNA rearrangements and deletions. This observation provoked the question: Could similarly active transposable elements be artificially leveraged to reproduce the natural phenomena of genome reorganization in a controlled setting? The researchers’ experiments decisively answered yes.
Within a span of just ten weeks, the engineered E. coli strains exhibited about 25 new insertion events of transposable elements coupled with genome size alterations exceeding 5%, either through expansion or contraction. This rate of genomic remodeling mirrors what would otherwise transpire naturally over decades. Intriguingly, the data revealed a dynamic interplay between frequent small deletions and rare but significant duplications, challenging the prevailing notion that genome reduction results from a simple bias toward deletion. Instead, transient expansions punctuate the evolutionary landscape, complicating previous binary models and underscoring the nuanced evolutionary choreography operating at the genomic level.
One of the most captivating revelations was the emergence of composite transposons—genetic elements formed when two or more ISs flank a piece of DNA, creating a mobile unit capable of shuttling genes around the genome. This finding provides valuable insights into potential evolutionary routes for the genesis and diversification of complex transposable elements. The rapid generation and fixation of these composite structures suggest a richer tapestry of genomic innovation mediated by mobile DNA than previously appreciated.
Beyond elucidating bacterial genome evolution, this platform offers an unparalleled experimental framework to assess the fitness consequences and adaptive significance of insertion sequence activity. The capacity to fine-tune genome size, arrangement, and composition through accelerated IS activation means that researchers can model evolutionary outcomes with remarkable precision, probing how structural changes affect cellular physiology, ecological interactions, and evolutionary potential. Such detailed mechanistic insights were hitherto constrained by the slow pace of natural evolution.
Reflecting on these far-reaching implications, Kanai emphasized that this technology also provides a window into the evolutionary behavior of the transposons themselves. While their role as genome architects is well-recognized, the evolutionary strategies and population dynamics underlying transposon proliferation and regulation have remained relatively obscure. The observed patterns of transposon activity, expansion, and structural rearrangement in the laboratory may illuminate the selective pressures, conflicts, and cooperative interactions shaping transposon evolution.
The accelerated evolutionary system invites bold new experiments addressing fundamental questions about microbial life and evolution. For instance, the team is eager to explore the environmental and genetic conditions that favor the emergence of cooperative interactions—either between bacterial strains or between bacteria and their hosts. Understanding such cooperative evolution could illuminate pathways to complex symbioses and microbial community dynamics, topics of profound relevance in ecology, medicine, and biotechnology.
Kanai envisions the broader horizon whereby this research contributes to a grand endeavor: decoding how biological complexity emerges from simpler life forms. By iteratively selecting for intricate genome reconfigurations and adaptive traits, it could soon be possible to engineer and evolve minimal yet sophisticated organisms in the laboratory. Such organisms might serve as models for the origins of complexity or as platforms for designing novel biological materials and systems with properties unattainable through direct rational design.
This work emerges from the confluence of molecular genetics, evolutionary biology, and synthetic biology, reflecting the power of integrative and interdisciplinary science. The team’s achievement redefines our capability to experimentally interrogate genome evolution, shifting from passive observation to active engineering of evolutionary trajectories. This not only accelerates scientific discovery but also heralds new technological frontiers in creating custom-designed life forms.
In summary, the University of Tokyo team’s innovative method, centered on amplifying high-activity insertion sequences within bacteria, transforms evolutionary study from retrospective analysis into a dynamic, real-time exploration of genome architecture changes. Overcoming the temporal constraints of natural evolution, their approach reveals the hidden layers of genome dynamics, highlighting processes manifold in scale and complexity. The implications stretch from microbiology and evolutionary theory to biotechnology and synthetic biology, promising a revolution in how we understand and utilize the evolutionary fabric of life.
As we stand on the cusp of this new era, the accelerating force of mobile genetic elements invites us to reconsider the evolutionary narrative itself—not as a slow and steady march but as a rapid, intricate dance of genetic innovation and reorganization. With further research building on these findings, the scientific community may soon fathom the foundational principles dictating how genomes evolve, diversify, and generate the complexity that defines life on Earth.
Subject of Research: Cells
Article Title: Laboratory evolution of the bacterial genome structure through insertion sequence activation
News Publication Date: 10-May-2025
Web References: doi.org/10.1093/nar/gkaf331
References: Yuki Kanai, Atsushi Shibai, Naomi Yokoi, Saburo Tsuru, Chikara Furusawa, “Laboratory evolution of the bacterial genome structure through insertion sequence activation,” Nucleic Acids Research, 2025.
Image Credits: ©2025 Kanai et al. CC-BY-ND
Keywords: bacterial genome evolution, insertion sequences, transposons, Escherichia coli, genome structure, mobile genetic elements, accelerated evolution, synthetic biology, genome rearrangement, evolutionary biology
Tags: accelerated bacterial genome evolutionbacterial evolution in the labexperimental evolutionary biology advancesgenome rearrangements in microorganismsgenomic complexity challengesinsertion sequences in E. colijumping genes in bacterialaboratory evolution experimentsmobile genetic elements researchreal-time observation of evolutionUniversity of Tokyo evolutionary biologyYuki Kanai research team
What's Your Reaction?
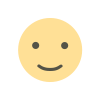
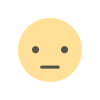
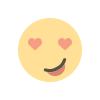
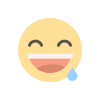
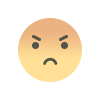
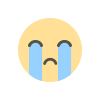
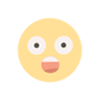