Proteins Essential for Healthy Brain Development Captured on Film
In the intricate and bustling environment of a cell, microscopic highways called microtubules facilitate the movement of essential cargo such as organelles, proteins, and genetic material. This intracellular transit system relies heavily on motor proteins, which transport their loads with precision and efficiency. Among these motor proteins, dynein stands out due to its unique role […]

In the intricate and bustling environment of a cell, microscopic highways called microtubules facilitate the movement of essential cargo such as organelles, proteins, and genetic material. This intracellular transit system relies heavily on motor proteins, which transport their loads with precision and efficiency. Among these motor proteins, dynein stands out due to its unique role in ferrying cellular components toward the cell’s center, specifically the nucleus. Understanding the detailed mechanisms that regulate dynein’s activation and function is crucial, as disruptions in this pathway have been implicated in severe neurodevelopmental and neurodegenerative diseases.
One particularly vital regulator of dynein is a protein called Lis1. Lis1’s partnership with dynein is essential for proper motor activity, and defects in Lis1 are known to cause lissencephaly, a rare but devastating brain development disorder commonly referred to as “smooth brain” due to its characteristic lack of normal brain folds. Despite the critical nature of this partnership, many aspects of how Lis1 activates dynein and modulates its function have remained mysterious. Now, groundbreaking research led by teams from the Salk Institute and the University of California, San Diego, has captured for the first time short, high-resolution movies revealing the stepwise activation of dynein by Lis1. These movies illustrate sixteen distinct structural conformations of dynein during its interaction with Lis1, providing unprecedented insight into the molecular dance that governs this essential cellular process.
At the molecular level, dynein is a large protein complex composed of two symmetrical halves. Each half includes a stalk domain, which binds to the microtubule; a tail domain, which attaches to the cargo; and a motor domain, which hydrolyzes ATP to generate movement. This ATP-driven motor domain functions somewhat like a biological engine, allowing dynein to “walk” along microtubule tracks. In its inactive state, dynein adopts a locked conformation known as the Phi state, in which it is unable to interact productively with the microtubule, effectively putting the motor “on pause” until activation signals prompt it otherwise.
Prior research had suggested that Lis1 plays the role of an activator or “key” that unlocks dynein, transforming it into an open, active conformation termed the Chi state. However, these conclusions were largely based on static images that captured only snapshots of the dynein-Lis1 interaction at isolated moments, leaving the full dynamic process poorly understood. The new study overcame this limitation by employing time-resolved cryogenic electron microscopy (cryo-EM), a cutting-edge imaging technique that collects a series of structural data points over fractions of a second to generate detailed 3D “movies” of molecular interactions.
Using yeast cells as a model system—chosen for their ability to withstand alterations in dynein and Lis1 levels and the high degree of conservation between yeast and human dynein—the researchers were able to isolate the dynein-Lis1 complex and dramatically lower the temperature to slow the protein motions. This approach made it possible to capture a series of high-definition 3D snapshots that, when combined, depict a continuous timeline from dynein’s locked Phi state through a series of intermediates to the fully activated Chi conformation.
The findings reveal a sophisticated, multi-step activation mechanism. In the first step, one half of the Lis1 dimer binds to the motor domain of dynein. This initial contact disrupts dynein’s locked conformation, “turning on” the motor by prompting a shape change that enhances ATP hydrolysis efficiency and primes the protein for movement. Subsequently, the second half of Lis1 binds to the stalk domain of dynein, further stabilizing the activated conformation and significantly boosting dynein’s motor activity. This two-tiered interaction not only unlocks dynein but also amplifies its capacity to harness ATP energy for rapid and directed transport along the microtubules.
The biological implications of these discoveries extend far beyond the realm of basic cellular biology. Lis1-associated dynein dysfunction is critical in the etiology of lissencephaly and other neurological disorders, which currently have no cure. By deciphering the precise molecular details of dynein activation, this research lays a foundation for rational drug design aimed at restoring proper motor function. For example, small molecules engineered to emulate Lis1’s activating effects on dynein could potentially counteract the impacts of Lis1 mutations or deficiencies.
Furthermore, the high-resolution cryo-EM data give researchers a detailed roadmap of potential binding sites for therapeutic compounds within the dynein-Lis1 complex. Mapping these targetable sites opens new avenues to develop precision medicines that modulate motor protein activity at the atomic level, providing hope for treating not only rare genetic brain disorders but potentially a broader range of neurodegenerative diseases where cellular transport pathways are compromised.
Looking forward, further investigations will focus on dissecting how specific mutations in Lis1 or dynein contribute to disease states by altering their interaction dynamics. Moreover, expanding these studies to human proteins and neuronal cell models will be critical in validating the therapeutic potential suggested by the yeast model findings. These efforts signify an important step toward bridging molecular insights with clinical applications.
This study exemplifies the power of advanced structural biology techniques like time-resolved cryo-EM in transforming our understanding of dynamic protein machines. By transforming still images into molecular movies, researchers can now witness the intricate choreography that underlies fundamental processes such as intracellular transport. The capacity to visualize molecular events as they unfold in near real-time will undoubtedly accelerate the discovery of novel treatments for complex diseases linked to protein dysfunction.
The research was conducted by a collaborative team including Agnieszka Kendrick of the Salk Institute, Andres Leschziner of UC San Diego, and colleagues such as Kendrick Nguyen, Eva Karasmanis, Rommie Amaro, Samara Reck-Peterson, and Wen Ma. Supported by prestigious funding bodies including the American Cancer Society, National Institutes of Health, the Cardiovascular Research Institute of Vermont, Jane Coffin Childs Postdoctoral Fellowship, and the Howard Hughes Medical Institute, this work underscores the importance of interdisciplinary collaboration in pushing the frontiers of biomedical science.
As a distinguished institution, the Salk Institute continues to advance the understanding of life’s most intricate mechanisms, from neuroscience to cancer, aging, and beyond. Founded by Jonas Salk, the architect of the first safe polio vaccine, the Institute embodies a legacy of dedication to uncovering biological truths with the potential to transform human health.
—
Subject of Research: Molecular mechanisms of dynein motor protein activation by Lis1 and implications for neurodevelopmental disorders.
Article Title: Multiple steps of dynein activation by Lis1 visualized by cryo-EM.
News Publication Date: May 23, 2025.
Web References: https://www.nature.com/articles/s41594-025-01558-w
References: Kendrick A., Leschziner A., et al. (2025). Multiple steps of dynein activation by Lis1 visualized by cryo-EM. Nature Structural & Molecular Biology. DOI: 10.1038/s41594-025-01558-w
Image Credits: Agnieszka Kendrick, Salk Institute
Keywords: Dynein, Lis1, motor proteins, microtubules, cryo-electron microscopy, neurodevelopmental disorders, lissencephaly, protein activation, intracellular transport, structural biology, ATP hydrolysis, neurodegeneration
Tags: brain development disorders understandingdynein motor protein functionhigh-resolution imaging in biologyintracellular transport systemsLis1 protein and brain developmentlissencephaly causes and effectsmicrotubules in cellular transportNeurodegenerative disease researchneurodevelopmental diseases mechanismsprotein interactions in cellsSalk Institute research findingsUC San Diego neuroscience studies
What's Your Reaction?
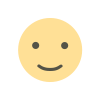
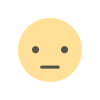
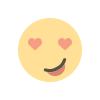
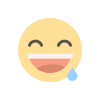
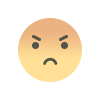
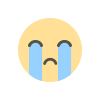
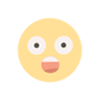