New World Record Set for Lithium-Ion Conductor Performance
In a groundbreaking advancement poised to redefine the limits of ionic conductivity in battery materials, researchers led by Professor Thomas F. Fässler at the Technical University of Munich (TUM) have unveiled a novel lithium antimonide compound engineered through precise structural manipulation. This innovative material demonstrates an unprecedented level of ionic transport efficiency, a development that […]

In a groundbreaking advancement poised to redefine the limits of ionic conductivity in battery materials, researchers led by Professor Thomas F. Fässler at the Technical University of Munich (TUM) have unveiled a novel lithium antimonide compound engineered through precise structural manipulation. This innovative material demonstrates an unprecedented level of ionic transport efficiency, a development that could catalyze the next generation of high-performance energy storage technologies. The collaborative effort married expertise in inorganic chemistry and electrochemistry to engineer a compound where lithium is partially substituted with scandium—a strategic intervention that introduces vacancies, or deliberate gaps, within the crystal lattice, fundamentally enhancing ionic mobility.
The essence of this breakthrough lies in the role of scandium ions within the crystal framework. By replacing lithium ions with scandium, the team effectively created controlled disruptions or vacancies in the lattice structure of Li3Sb. These vacancies serve as pathways, dramatically improving the diffusivity of lithium ions throughout the material. Ion mobility is a critical factor influencing the charge-discharge rates in lithium-ion batteries, and this vacancy engineering presents an elegant solution to overcome inherent conductivity barriers seen in conventional materials.
The standard lithium antimonide compound, while a known conductor, exhibited limitations in ion transport efficiency that hindered its practical application in battery components. Scandium substitution does not merely inject new atoms into the lattice but induces a form of structural disorder beneficial to ionic conductivity. This fine balance between maintaining crystal integrity and introducing functional disorder is at the heart of materials design for advanced ionic conductors, and the TUM team’s success reflects their meticulous synthesis and characterization techniques.
Validation of the extraordinary ionic conductivity observed was no straightforward endeavor, as the new material concurrently conducts electrons, a property that complicates traditional ionic conductivity measurements. To address this, the research group collaborated with the Chair of Technical Electrochemistry at TUM, under the direction of Professor Hubert Gasteiger. Using adapted and highly sensitive electrochemical methods, co-author Tobias Kutsch undertook rigorous assessments confirming that the material’s ionic conductivity substantially surpasses existing benchmarks. These developments suggest that conventional measurement paradigms need reassessment when addressing dual-conductive materials.
Beyond its remarkable conductivity, the scandium-doped lithium antimonide exhibits impressive thermal stability and can be synthesized using established chemical processes. Thermal robustness is critical for materials intended for battery electrodes, as operational temperatures often fluctuate and can degrade less stable compounds. The coupling of high ionic conductivity with dependable thermal characteristics positions this material as a promising candidate for real-world application, particularly as an additive to electrode architectures where accelerated ion transport can directly translate into improved battery performance.
The implications of this research extend far beyond a single compound. First author Jingwen Jiang from TUM’s Energy Research division highlights that while the immediate findings concern lithium-antimony systems, the underlying principle of vacancy engineering via targeted elemental substitution is transferable. Lithium-phosphorus systems, for instance, could also benefit from this approach, suggesting a broader paradigm shift. The prior art involved complex multi-element systems, such as lithium-sulfur compounds requiring the integration of five additive elements to optimize performance. In stark contrast, this work demonstrates that a single additional component—scandium—can induce superior ionic conductivity, simplifying material design and potential scalability.
Vacancy engineering in ionic conductors taps into a nuanced understanding of crystallographic principles and defect chemistry. Traditionally viewed as imperfections, vacancies in this context are deliberately introduced structural features that facilitate ionic transport by providing vacant sites that ions can hop into. This mechanism reduces energy barriers and accelerates ion diffusion—a core requirement for any material aspiring to improve battery charge rates and efficiency.
Patent filings underscore the innovative potential of this discovery, reflecting both academic and commercial interest in leveraging the material’s unique properties. As the research progresses from fundamental studies toward practical implementation, the team anticipates refining synthesis pathways and integrating the material into functional electrode matrices. Given that dual ionic and electronic conduction materials are particularly suited as conductive additives, they hold promise for enabling faster-charging and higher-capacity battery cells, which remain paramount objectives in energy storage research.
The research also sheds light on how subtle manipulations at the atomic scale can yield outsized improvements in macroscopic material properties. This resonates with a broader trend in materials science, where precision in chemical composition and crystallographic arrangement is increasingly harnessed to push the boundaries of performance in energy-related materials.
As the global push toward sustainable energy solutions intensifies, innovations like scandium-induced vacancy engineering exemplify the intersection of fundamental science and technological relevance. The scalability of producing such a material using established chemical methods further enhances its attractiveness, potentially facilitating seamless integration within existing manufacturing frameworks for lithium-ion batteries.
Professor Fässler’s team envisions that ongoing investigations will optimize the concentration of scandium substitution and fully elucidate the interplay between structural disorder and electrochemical performance. These studies will be pivotal for transitioning this discovery from laboratory curiosity to a staple component in next-generation batteries. If successful, this could substantially enhance electric vehicle range, reduce charging times, and ratchet up the overall performance of portable electronics—transforming everyday energy engagement.
In conclusion, this remarkable advancement redefines the conceptual framework for engineering high-performance ionic conductors by demonstrating that strategic elemental substitution and vacancy creation can spearhead leaps in conductivity unmatched by existing multi-element complex materials. As research intensifies, the battery industry and energy materials field alike watch closely, recognizing the potential ripple effects that this fundamental discovery promises.
Subject of Research: Not applicable
Article Title: Scandium Induced Structural Disorder and Vacancy Engineering in Li3Sb – Superior Ionic Conductivity in Li3−3xScxSbv
News Publication Date: 28-Apr-2025
Web References: 10.1002/aenm.202500683
Image Credits: Wenzel Schuermann / Technical University of Munich (TUM)
Keywords
lithium-ion conductivity, scandium substitution, vacancy engineering, lithium antimonide, ionic transport, battery materials, crystal lattice defects, electrochemical characterization, thermal stability, energy storage, inorganic chemistry, novel materials
Tags: breakthroughs in energy storage solutionscharge-discharge rates in lithium batterieselectrochemistry and inorganic chemistry collaborationenhanced ionic mobility in batterieshigh-performance energy storage technologiesionic conductivity advancementslithium antimonide compound innovationslithium-ion conductor performancenext-generation battery materialsscandium substitution in battery materialsstructural manipulation of ionic conductorsvacancy engineering in crystal structures
What's Your Reaction?
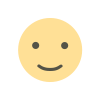
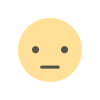
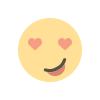
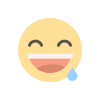
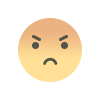
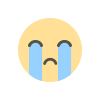
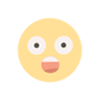