New Technique Quickly Measures Cell Density to Assess Health and Developmental Stage
CAMBRIDGE, MA — Understanding the intricate physical characteristics of cells has long been a frontier in biological research, with cell density emerging as a particularly revealing metric of cellular health and behavior. As cells undergo essential processes such as proliferation, differentiation, or programmed death, subtle shifts in their intracellular composition—water content, biomolecular crowding, and structural […]

CAMBRIDGE, MA — Understanding the intricate physical characteristics of cells has long been a frontier in biological research, with cell density emerging as a particularly revealing metric of cellular health and behavior. As cells undergo essential processes such as proliferation, differentiation, or programmed death, subtle shifts in their intracellular composition—water content, biomolecular crowding, and structural organization—manifest as measurable changes in density. Until recently, capturing these fine-scale variations at single-cell resolution across large populations was a formidable challenge, hampered by technical limitations in throughput and precision.
Now, a breakthrough from an interdisciplinary team at the Massachusetts Institute of Technology (MIT) promises to revolutionize how researchers and clinicians assess cellular states by enabling rapid, high-throughput single-cell density measurements. Leveraging cutting-edge microfluidic and optical technologies, this new technique can analyze up to 30,000 individual cells within just one hour, offering an unprecedented window into the dynamic physical properties that govern immune responses and cancer drug sensitivities.
Precise quantification of cell density offers an indirect but powerful snapshot of the molecular crowding within a cell’s cytoplasm and nucleus. Changes in density can reflect fluctuations in the abundance of lipids, proteins, nucleic acids, and, critically, water content. These shifts correlate with functional transitions, such as T cell activation or tumor cell susceptibility to chemotherapeutic agents. By carefully measuring these physical parameters with exceptional sensitivity and scale, the MIT team has unlocked the potential for new diagnostic and predictive biomarkers that complement traditional molecular analyses.
The foundation of this method builds on seminal work from MIT’s Manalis laboratory, which over the last two decades has pioneered the suspended microchannel resonator (SMR) technology. This microfluidic device utilizes a cantilever with an embedded microchannel that vibrates at specific frequencies. As a single cell flows through, the change in vibration frequency corresponds directly to its buoyant mass—a measure influenced by the cell’s mass and the density of the surrounding fluid. The frequency shift allows researchers to calculate the cell’s mass with extraordinary precision.
Previous iterations of this technology required cells to pass through the resonator multiple times suspended in fluids of varying densities to derive cell volume, and thereby calculate density. Although successful, this approach significantly constrained throughput, limiting its application to roughly a few hundred cells per experiment due to the complexity and temporal demands of fluid swapping.
Addressing these constraints, the MIT researchers have ingeniously integrated the SMR device with a fluorescence microscopy system that accurately measures cell volume in real time. By staining the suspension fluid with a fluorescent dye impermeable to cell membranes, the passage of each cell creates a transient dip in fluorescence intensity that corresponds to the cell’s volume. This volumetric information, combined with the subsequent resonator-based mass measurement, enables rapid calculation of cell density as the cells flow through the device in a continuous, one-pass manner. This streamlined methodology dramatically increases throughput without compromising measurement accuracy or sensitivity.
The implications of this technological advance extend deeply into immunology. Using this system, the team investigated the density dynamics of T cells as they transition from a resting quiescent state to an active, proliferative one triggered by antigenic stimulation. Remarkably, they observed a decrease in average cell density from approximately 1.08 grams per milliliter to 1.06 grams per milliliter within the first day post-activation. This reduction indicates an influx of water into the cells, causing them to swell and become less crowded with biomolecules. These findings provide compelling evidence that changes in cellular water content—and thus density—serve as meaningful biomarkers of immune cell fitness and functionality.
This functional relevance of density measurements holds clinical promise. The startup company Travera, co-founded by Professor Scott Manalis, has leveraged SMR technologies to predict the responsiveness of individual patients’ T cells to immunomodulatory drugs aimed at eliciting potent anti-tumor effects. Preliminary investigations reveal that combining cell mass and density measurements markedly enhances the predictive accuracy of immune cell competency, signaling a new path for personalized immunotherapy monitoring.
Beyond immune cells, the utility of this approach is underscored in oncology research. The MIT team applied their platform to pancreatic cancer cell lines exposed to chemotherapeutic agents with distinct efficacy profiles. They demonstrated that density changes following treatment were strongly indicative of cellular responses—distinguishing drug-susceptible cells undergoing apoptosis from resistant populations. These observations suggest that cell density measurements facilitate rapid, non-invasive predictions of tumor drug sensitivity, potentially informing precision oncology decisions within days.
Looking forward, Professor Manalis’ laboratory is expanding applications of these mass-density markers to optimize the production of complex biologics such as therapeutic antibodies. By monitoring the fitness and metabolic state of producer cells, this technique may enable real-time quality control and process refinement, advancing biomanufacturing efficiency and therapeutic yield.
The convergence of microfluidics, resonant sensing, and fluorescence microscopy manifests in a remarkable fusion of physical and biological insight. The ability to measure subtle, rapid changes in single-cell density at high throughput bridges a longstanding gap between physical biophysics and practical biomedical applications. It is a compelling example of how engineering innovations can uncover new dimensions of cell biology, with potential impacts ranging from immunotherapy to drug development and beyond.
Funded through support by the Paul G. Allen Frontiers Group, the Virginia and Daniel K. Ludwig Fund for Cancer Research, MIT’s Center for Precision Cancer Medicine, the Stand up to Cancer Convergence Program, Bristol Myers Squibb, and the National Cancer Institute’s Koch Institute Support Grant, this multidisciplinary effort highlights collaborative investment in technologies poised to transform biomedical research landscapes.
The publication of this study, led by MIT research scientist Weida (Richard) Wu and senior author Professor Scott Manalis, in the prestigious journal Nature Biomedical Engineering, marks a significant milestone in cell measurement science. It offers the scientific community a robust new tool to rapidly profile and predict cellular states, with broad ramifications for diagnostics, therapeutics, and industrial biotechnology.
As the field moves forward, the integration of high-throughput physical measurements promises not only to complement genomic and proteomic profiling but also to reveal new layers of cellular heterogeneity and function. By quantifying biophysical properties that have hitherto been difficult to access at scale, researchers are gaining a richer, more nuanced understanding of cell biology—one that could fundamentally reshape disease treatment paradigms and personalized medicine strategies worldwide.
Subject of Research: Cell density measurement and its application to immune cell activation and cancer drug response.
Article Title: High-throughput single-cell density measurements enable dynamic profiling of immune cell and drug response from patient samples
News Publication Date: 20-May-2025
Web References: 10.1038/s41551-025-01408-6
Keywords: Cancer, Cell density, Cell proliferation, Immune cells, Cancer cells, Cell biology, Diseases and disorders, Health and medicine
Tags: biomolecular crowding effectscancer drug sensitivity assessmentcell density measurement techniquecellular health evaluation methodsdevelopmental stage assessment in cellshigh-throughput cell assessmentimmune response analysisintracellular composition analysismicrofluidic technology in biologyMIT breakthrough in cellular researchoptical techniques for cell measurementsingle-cell analysis technology
What's Your Reaction?
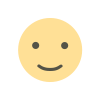
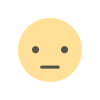
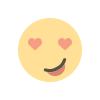
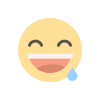
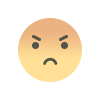
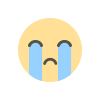
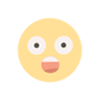