Native Nucleosomes Shape Genome Organization Intrinsically
In a groundbreaking study published in Nature, researchers have unveiled new insights into the fundamental forces driving genome organization, highlighting the intrinsic roles of nucleosomes and multivalent ions in promoting chromatin condensation. This research advances our understanding of how the genome’s three-dimensional architecture emerges from the interplay between biochemical modifications and electrostatic interactions, shedding light […]

In a groundbreaking study published in Nature, researchers have unveiled new insights into the fundamental forces driving genome organization, highlighting the intrinsic roles of nucleosomes and multivalent ions in promoting chromatin condensation. This research advances our understanding of how the genome’s three-dimensional architecture emerges from the interplay between biochemical modifications and electrostatic interactions, shedding light on the mechanisms underlying chromosome compartmentalization.
Polyamines, long suspected to compact DNA by bridging negative charges along the phosphate backbone, have been confirmed to induce extensive DNA and nucleosome condensation through robust charge–charge interactions. Using a novel high-resolution technique known as condense-seq, the team systematically compared the effects of various ionic condensing agents—including spermidine, cobalt hexamine, magnesium, and calcium ions—on native mononucleosomes derived from human embryonic stem cells. Their findings strikingly reveal that with the notable exception of calcium ions, all multivalent ions produce consistent condensation patterns at both megabase and kilobase scales, reinforcing the central role of electrostatic forces in chromatin organization.
Notably, the condensation profiles driven by these ionic agents consistently anticorrelate with gene expression levels across chromosomes, indicating that less transcriptionally active regions tend to be more compacted. This observation aligns with previous epigenomic data showing that repressive genomic compartments exhibit higher condensation. Interestingly, the poor condensation by calcium ions suggests a nuanced specificity of ionic species in modulating chromatin structure, possibly linked to their distinct charge densities and coordination properties.
Delving deeper into the molecular basis for nucleosome–nucleosome attraction, the researchers explored mutations on the acidic patch of the histone H2A/B dimer—a region previously implicated as a key interaction interface between nucleosomes. Charge swap mutations in this domain led to the most pronounced increase in condensability across the entire post-translational modification (PTM) library tested. This result underscores the importance of electrostatically mediated nucleosome proximity and provides compelling evidence that histone charge landscapes are pivotal determinants of chromatin compaction.
Complementing experimental data with sophisticated polymer simulations, the study demonstrated that nucleosome condensability alone suffices to predict the emergence of large-scale chromosomal A/B compartments, which typify active and inactive genome regions, respectively. This theoretical validation adds weight to the hypothesis that electrostatic interactions governed by nucleosome surface chemistry and ion-mediated bridging underlie the hierarchical organization of the genome.
The research also harnessed condense-seq to assess how non-ionic protein factors, specifically heterochromatin protein 1 isoforms HP1α and HP1β, influence chromatin condensation. Unlike ionic condensing agents, HP1 proteins elicited condensability profiles that, while still generally anticorrelated with gene expression on broader scales, diverged significantly at finer genomic resolutions. This divergence hints at distinct mechanistic pathways by which protein readers modulate chromatin structure compared to purely electrostatic condensation.
More strikingly, HP1-mediated condensation correlated tightly with nuclear subcompartment markers characteristic of heterochromatin, such as H3K9me3 histone methylation, a well-known hallmark of transcriptionally silent chromatin. These correlations illuminate the role of HP1 in reinforcing repressive chromatin architecture, consistent with its established function as an epigenetic “reader” protein that recognizes methylated histone tails to recruit silencing machinery.
Intriguingly, analyses revealed that while condensability at genetic promoters and enhancers was universally low for all condensing agents, this reduction was markedly less severe with HP1. This finding suggests that HP1-bound chromatin may retain a chromatin state more permissive to certain regulatory activities, juxtaposed against the more stringent compaction effects induced by ionic species. Additionally, HP1’s condensation effect diminished within gene bodies of highly expressed genes, contrasting with the pronounced condensation by ionic agents extending into these regions.
The study further demonstrated that the PTM landscape exerts profound influence over HP1-driven condensation. Specifically, nucleosomes marked with H3K9 trimethylation dramatically enhanced HP1α-induced condensation, solidifying the canonical link between this modification and heterochromatin formation. Unexpectedly, modifications on the H3 tail—regardless of type—also modestly boosted HP1-mediated condensation, particularly at sites distal from the nucleosome core, hinting at potential nonspecific interactions or indirect effects on nucleosome dynamics that facilitate HP1 binding.
In comparison to ionic agents, most PTMs outside the H3 tail affected condensability similarly for both HP1α and ionic condensing agents, typically reducing the tendency for condensation. These data collectively underscore a complex code where electrostatic forces, protein interactions, and histone modification states integrate to sculpt chromatin architecture.
This extensive characterization importantly advances the conceptual framework for understanding chromatin organization not as a static scaffold but as a dynamic, multivalent interaction network modulated by both biochemical cues and physical forces. The implications of these findings extend from fundamental genome biology to the pathological misregulation observed in cancers and developmental disorders linked to chromatin misfolding.
Beyond the mechanistic revelations, the innovative use of condense-seq provides a powerful platform to dissect chromatin dynamics at unprecedented molecular resolution, opening avenues for probing how environmental cues and cellular signaling influence genome topology through alterations in nucleosome physicochemical properties.
As research continues to decode the epigenomic and biophysical language of chromatin, studies like this pave the way for novel interventions that target genome organization in disease states. By harnessing the interplay between nucleosome charge patterning and multivalent ions, future therapeutic strategies might aim to modulate genome compartmentalization selectively, offering refined genomic control not attainable by conventional approaches.
Ultimately, this work cements a paradigm wherein the intrinsic electrostatic landscape of nucleosomes, shaped by histone modifications and ion-mediated bridging, encodes the spatial blueprint of the human genome. It challenges researchers to rethink chromatin not merely as a collection of DNA and proteins but as an electrostatically active polymer network whose architecture is finely tuned to cellular states and functions.
Subject of Research: Genome organization driven by nucleosome electrostatics and chromatin condensation mechanisms.
Article Title: Native nucleosomes intrinsically encode genome organization principles.
Article References:
Park, S., Merino-Urteaga, R., Karwacki-Neisius, V. et al. Native nucleosomes intrinsically encode genome organization principles. Nature (2025). https://doi.org/10.1038/s41586-025-08971-7
Image Credits: AI Generated
Tags: chromatin condensation studieschromosome compartmentalization insightselectrostatic interactions in genomicsepigenomic data correlationsgene expression and chromatin structuregenome organization mechanismshigh-resolution condense-seq techniqueionic agents in DNA compactionnative mononucleosomes researchnucleosome dynamicspolyamines and DNA interactiontranscriptional activity and genome architecture
What's Your Reaction?
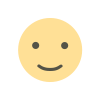
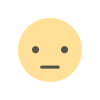
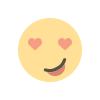
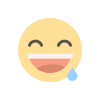
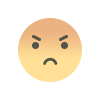
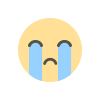
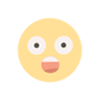