MINFLUX Nanoscopy Boosted by High-Order Vortex Beams
In the relentless pursuit of pushing optical microscopy beyond traditional limits, a groundbreaking advancement has emerged that promises to revolutionize nanoscale imaging. Researchers XJ Tan and Z. Huang have recently unveiled a novel enhancement to MINFLUX nanoscopy, employing high-order vortex beams to significantly elevate imaging resolution and precision. Published in the prestigious journal Light: Science […]

In the relentless pursuit of pushing optical microscopy beyond traditional limits, a groundbreaking advancement has emerged that promises to revolutionize nanoscale imaging. Researchers XJ Tan and Z. Huang have recently unveiled a novel enhancement to MINFLUX nanoscopy, employing high-order vortex beams to significantly elevate imaging resolution and precision. Published in the prestigious journal Light: Science & Applications in 2025, this new methodology stands to redefine the capabilities of super-resolution microscopy, opening unprecedented avenues in biological and materials science research.
MINFLUX, a revolutionary fluorescence nanoscopy technique introduced in the past decade, has already shattered conventional barriers by combining fluorescence detection with minimal photon fluxes to localize single molecules with nanometer precision. By illuminating a sample with a donut-shaped excitation light and analyzing the emitted fluorescence photons’ intensity variations, MINFLUX attains localization accuracy far superior to standard microscopy techniques. Still, the exact spatial confinement of the excitation light remains a limiting factor. Herein lies the transformative potential of incorporating high-order vortex beams, which have a complex phase structure and can carry orbital angular momentum.
High-order vortex beams differ fundamentally from the typical Gaussian beams employed in many conventional microscopy setups. They possess a helical wavefront characterized by phase singularities that manifest as dark cores surrounded by bright rings of light. This unique intensity and phase distribution enables enhanced manipulation of excitation light patterns, crucial for improving MINFLUX’s spatial resolution. Addressing the challenges in precisely steering and shaping these vortex beam profiles has allowed Tan and Huang to tailor MINFLUX’s illumination in unprecedented ways.
The new approach effectively uses high-order vortex beams to engineer the fluorescence excitation pattern at the nanoscale, creating more intricate and sharply confined light distributions. By fine-tuning the vortex beam order, the researchers manipulate the size and intensity profile of the donut beam’s central dark spot, achieving sharper intensity gradients. These gradients directly improve the localization accuracy of the fluorescent emitters by enhancing the contrast in the emitted signal with respect to their position. This refined control over the excitation geometry allows MINFLUX to more precisely pinpoint molecular locations even in densely labeled biological environments.
A paramount challenge in deploying high-order vortex beams arises from their sensitivity to optical aberrations and their propensity to distort upon propagation through complex media. Tan and Huang’s work overcomes this by incorporating adaptive optics and advanced beam-shaping techniques, ensuring stable and reproducible beam profiles within the imaging system. Their setup leverages spatial light modulators (SLMs) to dynamically generate and adjust the vortex beam modes in real-time, adapting to sample-induced distortions and maintaining diffraction-limited focusing. This dynamic flexibility enhances the robustness and practical applicability of the enhanced MINFLUX setup.
Furthermore, the integration of high-order vortex beams leads to a substantial reduction in photobleaching and phototoxicity during imaging. Since MINFLUX fundamentally minimizes the number of excitation photons needed for localization, sharpening the spatial excitation with vortex beams further concentrates photon delivery precisely where needed. This spatiotemporal photon economy preserves fluorophore integrity and prolongs sample viability—critical considerations in live-cell imaging and long-term observation of dynamic biological processes.
The implications of this technological leap extend markedly into cellular and molecular biology realms. By resolving fluorophores with sub-nanometer accuracy in complex, densely packed intracellular environments, researchers can now track biomolecular interactions and protein dynamics with unparalleled clarity. Processes such as synaptic vesicle trafficking, receptor clustering on cell membranes, and DNA-protein interactions can be visualized in vivo with spatial detail and temporal fidelity previously thought unattainable through optical microscopy.
Tan and Huang’s concept also paves the way for synergistic combinations with complementary super-resolution techniques like STED and PALM, potentially amalgamating strengths in photon efficiency, resolution, and multiplexing ability. Moreover, the use of vortex beams carrying orbital angular momentum opens doors for encoding additional information channels into the excitation light, offering prospects for multi-dimensional imaging schemes that simultaneously probe structural, dynamic, and mechanical properties of nanoscale specimens.
On a fundamental level, this work underscores the profound influence of beam engineering on optical microscopy’s future. While the past decades witnessed incremental improvements by optimizing fluorophores or detection schemes, this study highlights the transformative value of fundamentally redefining how light’s phase and intensity distributions are harnessed. Employing sophisticated vortex beam modes with MINFLUX illustrates a new paradigm in microscope illumination optics, blending quantum optics principles with photonic engineering to transcend classical imaging constraints.
Technically, the setup developed involves an intricate alignment of laser sources, SLMs, and high-numerical-aperture objectives integrated within a feedback-controlled platform to stabilize vortex beam generation. Fluorescence signals are detected by single-photon avalanche diodes with real-time localization algorithms adapted to exploit the sharper spatial excitation profile. Calibration procedures include scanning nanostructured samples to meticulously characterize the point spread function alterations introduced by the high-order vortex modes.
This research also contributes valuable insights into beam-matter interactions at the nanoscale, particularly interactions involving complex field distributions. The controlled phase singularities and orbital angular momentum transferred from high-order vortex beams can influence fluorophore excitation dynamics and photophysics, aspects that Tan and Huang’s team have meticulously analyzed. Understanding these effects not only improves imaging fidelity but also informs the design of novel fluorescent probes tuned to respond to structured light excitation environments.
Importantly, the experimental validation involved imaging biological specimens labeled with conventional organic dyes and photoactivatable fluorescent proteins, verifying that the enhanced MINFLUX method achieves localization precisions approaching single-digit nanometers. Comparative analyses demonstrate marked resolution improvements compared to standard MINFLUX approaches, with the added benefits of decreased imaging times and reduced photodamage. This performance leap promises to accelerate investigations in systems biology, neuroscience, and nanomedicine.
Looking ahead, integrating artificial intelligence and machine learning with high-order vortex beam MINFLUX could further optimize beam patterns, compensate for system aberrations, and enhance real-time data interpretation. Such computational assistance might unlock adaptive imaging schemes where excitation profiles are dynamically tailored to specific sample features or biological events, maximizing information extraction while conserving sample integrity.
In conclusion, Tan and Huang’s innovative fusion of MINFLUX nanoscopy with high-order vortex beams addresses longstanding limitations in single-molecule localization microscopy. By harnessing the unique spatial and phase characteristics of vortex light, they have engineered an optical platform that elevates resolution, specificity, and sample compatibility to new heights. This advancement stands as a landmark achievement, poised to become a foundational tool in the expanding arsenal of nanoscale optical imaging technologies with far-reaching implications for scientific discovery and biomedical innovation.
Subject of Research:
MINFLUX nanoscopy enhancement using high-order vortex beams for improved super-resolution fluorescence imaging.
Article Title:
MINFLUX nanoscopy enhanced with high-order vortex beams.
Article References:
Tan, XJ., Huang, Z. MINFLUX nanoscopy enhanced with high-order vortex beams. Light Sci Appl 14, 184 (2025). https://doi.org/10.1038/s41377-025-01822-0
Image Credits:
AI Generated
DOI:
https://doi.org/10.1038/s41377-025-01822-0
Tags: biological imaging innovationscomplex phase structures in opticsfluorescence detection techniqueshigh-order vortex beamsimaging resolution enhancementmaterials science research applicationsMINFLUX nanoscopynanoscale imaging advancementsorbital angular momentum in opticsphoton flux minimizationsuper-resolution microscopytransformative microscopy methodologies
What's Your Reaction?
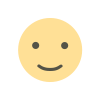
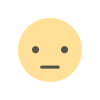
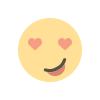
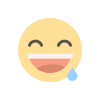
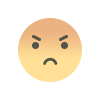
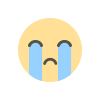
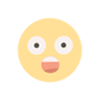