Light-Activated DNA G-Quadruplex Controls Gene Expression
In a groundbreaking advancement that promises to revolutionize the way we manipulate genetic activity, researchers have unveiled a novel method to control gene expression with unprecedented precision using light. The study, spearheaded by Zhang, X., Dhir, S., Melidis, L., and colleagues, and published in Nature Chemistry in 2025, introduces a DNA G-quadruplex-targeting reversible photoswitch, a […]

In a groundbreaking advancement that promises to revolutionize the way we manipulate genetic activity, researchers have unveiled a novel method to control gene expression with unprecedented precision using light. The study, spearheaded by Zhang, X., Dhir, S., Melidis, L., and colleagues, and published in Nature Chemistry in 2025, introduces a DNA G-quadruplex-targeting reversible photoswitch, a molecular innovation that could pave the way for dynamic, non-invasive gene regulation technologies.
At the heart of this research lies the G-quadruplex (G4) DNA structure. Unlike the canonical double helix most are familiar with, G4s are unique four-stranded configurations formed by guanine-rich sequences within the genome. These structures have long been implicated in key cellular processes such as transcription, replication, and genomic stability. Their presence in promoter regions and untranslated regions of genes makes them compelling targets for therapeutic intervention and precise gene regulation.
The scientists have ingeniously designed a photoswitchable molecule that selectively binds to these G-quadruplex structures and modulates their conformation in response to specific wavelengths of light. This reversible binding mechanism allows for spatial and temporal control over gene expression, enabling researchers to ‘switch on’ or ‘switch off’ gene activity in living cells by simply shining light of the appropriate color.
One of the most exciting aspects of this discovery is the reversible nature of the system. Traditional approaches to gene regulation—like genetic knockouts or chemical inhibitors—are often irreversible or lack real-time controllability. By integrating photoswitchable compounds that cycle between distinct configurations upon irradiation, the researchers have effectively created a genetic dimmer switch. This novel toolkit affords unparalleled flexibility, offering the ability to fine-tune gene expression dynamically, with high temporal resolution.
The photoswitch utilized in this study is based on azobenzene derivatives, molecules known for their light-induced reversible isomerization between trans and cis forms. By tailoring the chemical framework of the azobenzene moiety, the team achieved optimized binding affinity and specificity for G4 DNA, ensuring that off-target interactions are minimized. Light wavelengths in the visible range prompt structural transformations within the photoswitch without causing significant photodamage to the cells, enhancing the system’s biocompatibility.
Experimental validation involved complex in vitro assays to characterize binding kinetics, melting temperature shifts of G-quadruplexes upon photoswitch interaction, and gene expression analyses in cultured cells. The researchers demonstrated that upon irradiation with one light wavelength, the photoswitch stabilizes the G4 structure, impeding transcription factor binding and downregulating target gene expression. Conversely, exposure to an alternate wavelength induces photoswitch isomerization, relaxing the G4 conformation and restoring gene transcription.
This dual-wavelength control mechanism allows investigators to harness light—a clean, non-invasive stimulus—to regulate gene expression with unmatched agility and reversibility. Such precise control opens new vistas in functional genomics, enabling the study of gene activity kinetics and the dissection of complex regulatory networks under physiological conditions.
Beyond research laboratories, the implications for medicine and biotechnology are profound. The ability to remotely and reversibly modulate specific genes holds promise for developing next-generation gene therapies, tissue engineering protocols, and synthetic biology circuits. For instance, disease-associated genes could be targeted and silenced when necessary and reactivated as patient conditions evolve, all via externally applied light pulses.
Furthermore, the spatial precision afforded by light means that gene expression can potentially be manipulated in specific tissues or even single cells within an organism. This selectivity mitigates the risks associated with systemic drug treatments or genetic modifications that affect the entire body, heralding a new era of precision therapeutics.
One hurdle in phototherapy has traditionally been light penetration through biological tissues. However, the team overcame this limitation by engineering photoswitches responsive to red and near-infrared light, wavelengths that penetrate deeper into tissues with minimal scattering and absorption. This strategic choice broadens the platform’s applicability, including possible in vivo implementations in mammalian models and therapeutic contexts.
The study also conducted extensive toxicity assays to confirm that the photoswitch compounds and their light activation cycles do not induce cytotoxicity or genomic instability. These findings ensure that the system can be used safely in experimental and potentially clinical settings, alleviating concerns about off-target or deleterious effects during repeated activation cycles.
From a synthetic chemistry standpoint, the modular design strategy employed for the photoswitch facilitates further functionalization and tuning. This adaptability means that future iterations could incorporate targeting ligands, fluorescent reporters, or additional control domains, thereby enhancing specificity and enabling multiplexed regulation of numerous genes simultaneously.
Moreover, the use of DNA G-quadruplexes as scaffolds for photoswitchable intervention underscores the versatility of non-canonical DNA structures as regulatory nodes. It invites a re-examination of other such unconventional DNA architectures—like i-motifs or triplexes—as potential platforms for optogenetic or chemogenetic control strategies.
The authors envision that this technology could be integrated with existing optogenetic and nanotechnological approaches, forging powerful hybrid systems for controlling cellular behavior. For example, coupling photoswitchable G4 binders with light-responsive delivery vehicles or CRISPR-based effectors might exponentially increase the precision and scope of genetic modulation achievable in living organisms.
This innovation aligns well with the burgeoning field of synthetic biology, where dynamic control over gene circuits is essential. The ability to turn gene expression on and off within hours or even minutes, reversibly and without permanent undertakings, will significantly enhance our understanding of gene function and cellular responses.
Looking forward, the research community anticipates expanding the photoswitch toolset to target a broader repertoire of G4 sequences throughout the genome, including oncogene promoters, telomeres, and viral genomes. Such expansion could enable new interventions in cancer, aging, and infectious diseases.
While the conceptual groundwork and initial demonstrations are compelling, several challenges remain. Effective delivery of photoswitch molecules into target tissues in vivo, precise dosimetry of light exposure, and potential immunogenic responses to novel compounds must be thoroughly explored before clinical translation.
Nevertheless, this pioneering study delineates an exciting strategy for gene modulation at the intersection of chemistry, molecular biology, and photophysics. By marrying reversible photoswitches with G-quadruplex targeting, Zhang and colleagues have opened a promising pathway toward harnessing light as the ultimate remote control for the genome.
The implications stretch far beyond gene regulation, as the principles underlying this approach may inform the design of light-controlled epigenetic modifiers, RNA regulators, and protein activity modulators. This holistic control over biological systems at multiple regulatory levels could redefine the future of precision medicine and biotechnological innovation.
In essence, as we continue to decode the language of life, the ability to interact with this code using nothing more than light offers a powerful, elegant, and minimally invasive modality—ushering in a new dawn where biological functions can be switched on and off with the flick of a photon.
Subject of Research: Optical control of gene expression via DNA G-quadruplex targeting reversible photoswitch.
Article Title: Optical control of gene expression using a DNA G-quadruplex targeting reversible photoswitch.
Article References:
Zhang, X., Dhir, S., Melidis, L. et al. Optical control of gene expression using a DNA G-quadruplex targeting reversible photoswitch. Nat. Chem. (2025). https://doi.org/10.1038/s41557-025-01792-1
Image Credits: AI Generated
Tags: DNA G-quadruplex technologydynamic gene regulation methodsguanine-rich DNA structuresinnovative gene expression modulationlight-activated gene expression controlmolecular innovations in biotechnologyNature Chemistry publication on gene controlnon-invasive genetic manipulationphotoswitchable molecules in geneticsreversible photoswitch for gene regulationspatial and temporal gene controltherapeutic gene regulation techniques
What's Your Reaction?
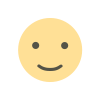
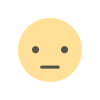
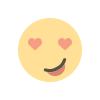
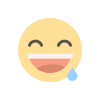
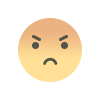
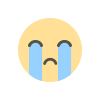
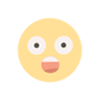