Graz University of Technology Researchers Unravel Heat Conduction in Complex Materials
In recent years, the study of organic semiconductors has gained substantial attention due to their potential applications in a variety of fields, such as organic light-emitting diodes (OLEDs) and solar energy conversion. However, understanding the thermal transport mechanisms within these complex materials has, until recently, been significantly overlooked. Researchers at Graz University of Technology, in […]

In recent years, the study of organic semiconductors has gained substantial attention due to their potential applications in a variety of fields, such as organic light-emitting diodes (OLEDs) and solar energy conversion. However, understanding the thermal transport mechanisms within these complex materials has, until recently, been significantly overlooked. Researchers at Graz University of Technology, in collaboration with esteemed institutions like TU Vienna and the University of Cambridge, have made groundbreaking strides in this area, driving forward our comprehension of how heat moves through organic semiconductors. This pioneering effort opens the doorway to designing materials with tailored thermal properties that could enhance their efficiency for various applications.
The quest to decipher thermal transport in organic semiconductors is both remarkable and intricate. Historically, the scientific focus has predominantly centered around charge transport, leaving researchers with a conspicuous gap in knowledge regarding how thermal energy is managed within these materials. According to Egbert Zojer, a prominent physicist leading the research, this research trajectory aimed to build a bridge between understanding both charge and thermal transport. The knowledge gleaned from this endeavor could significantly impact future innovations in organic materials, which are increasingly coveted in technological applications.
One of the most intriguing aspects of this study is the application of machine learning to decipher the intricacies of heat transport. Traditional approaches have relied heavily on empirical observations, causing researchers to miss out on potential causal connections within the materials. In this case, however, the research team opted to pursue a more fundamental path, striving for a deeper understanding of the underlying mechanics governing their thermal behaviors. By leveraging machine-learned potentials, they meticulously analyzed the distribution of heat in organic semiconductors, confronting conventional models that exclusively attributed thermal transport to the behavior of phonons.
Phonons, which represent quantized modes of vibration within a crystal lattice, have traditionally been treated as particles responsible for carrying vibrational energy. However, the research team’s findings suggest that a more intricate mechanism is at play. They uncovered evidence of tunneling transport, an additional phenomenon whereby phonons exhibit wave-like characteristics, communicating across energetic barriers within the solid matrix. This nuanced understanding of thermal transport radically reshapes the classical viewpoints surrounding the conductivity of materials, urging the scientific paradigm to embrace this more comprehensive perspective.
Equally noteworthy is the discovery that the molecular length of organic semiconductors plays a critical role in heat transport efficiency. The research team revealed that larger molecular sizes enhance tunneling effects, profoundly altering the thermal conductivity of these materials. This correlation introduces a new dimension to material design, where scientists can strategically manipulate molecular structures to optimize thermal transport tailored for specific applications. For example, in scenarios where achieving a high thermoelectric effect is crucial, focus can shift to promoting low thermal conductivity, whereas other applications may demand enhanced thermal dissipation capabilities.
Moreover, the research team’s insights extend well beyond just organic semiconductors. They propose that these findings could also be relevant to the design of metal-organic frameworks (MOFs), a class of materials recognized for their versatility and potential applications spanning from gas storage to catalysis. The intricate interplay of heat transport within MOFs makes this research invaluable, as the capability to manipulate heat conduction could significantly enhance the efficiency of various applications, paving the way for advanced innovations.
As researchers delve deeper into the mechanisms of thermal energy transport, it is clear that this study will catalyze an evolution in how materials are engineered. The traditional constraints confining our understanding of heat transfer are being dismantled, leading to new possibilities for scientists to tailor the thermal properties of materials graphically and purposefully. This transformative approach has the potential to revolutionize the fabrication of organic semiconductors and MOFs, creating opportunities for exponentially more efficient technologies in energy harvesting, electronics, and beyond.
In summary, the strides made by Egbert Zojer and his research team signify a monumental leap in the fields of materials science and thermodynamics. By integrating machine learning with fundamental research in thermodynamics, they have unraveled a newfound understanding of how heat travels in organic semiconductors which can profoundly reshape material design strategies. This research not only addresses a long-standing mystery but also illuminates a pathway toward discovering and engineering next-generation materials acclimatized to the demands of modern technology. As scientists worldwide continue to explore the implications of these findings, the future of organic semiconductors and thermal management appears more promising than ever.
This work was published in the highly regarded journal npj Computational Materials, calling attention to the importance of computational modeling in contemporary research. The merging of computational simulations with physical experiments underscores a prevailing trend: the reliance on advanced computing capabilities to tackle complex scientific challenges. As more researchers embrace these innovative methodologies, we can expect the pace of discoveries in materials science to accelerate, propelling forward our quest to harness nature’s fundamental principles for technological advancement.
Within academia and industrial circles alike, the excitement generated by these findings is palpable. The potential to utilize these principles for practical applications—be it in enhancing solar cell efficiency, creating advanced thermal insulating materials, or improving electronic devices—means that this research has implications that stretch far beyond theoretical interest, carving a niche for sustainable technology solutions that could enrich societal advancements in the years to come.
Subject of Research: Heat transport in crystalline organic semiconductors
Article Title: Heat transport in crystalline organic semiconductors: coexistence of phonon propagation and tunneling
News Publication Date: 14-Feb-2025
Web References: 10.1038/s41524-025-01514-8
References: npj Computational Materials
Image Credits: Credit: Lunghammer – TU Graz
Keywords
Organic semiconductors, thermal transport, machine learning, phonon propagation, tunneling transport, molecular length, metal-organic frameworks, material design, thermoelectric effect, computational modeling, energy efficiency
Tags: advancements in organic materialscharge and thermal transport relationshipcollaboration in materials scienceGraz University of Technology researchheat conduction mechanismsinnovative applications of organic semiconductorsOLED efficiency improvementsorganic semiconductorssolar energy conversion technologiestailored thermal properties in materialsthermal transport in materialsunderstanding complex materials in physics
What's Your Reaction?
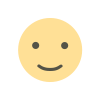
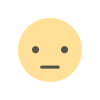
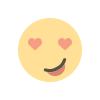
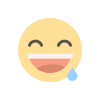
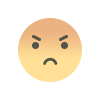
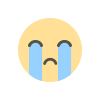
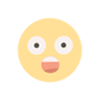