Graphite-Protected Organic Layers Boost Solar Water Splitting
In a groundbreaking advance poised to reshape the landscape of solar fuel generation, a team of researchers has unveiled organic photoactive layers capable of setting new benchmarks in solar water oxidation and unassisted water splitting. Their work, recently published in Nature Energy, demonstrates that graphite-protected bulk heterojunction organic photoactive layers can achieve unprecedented photocurrent densities […]

In a groundbreaking advance poised to reshape the landscape of solar fuel generation, a team of researchers has unveiled organic photoactive layers capable of setting new benchmarks in solar water oxidation and unassisted water splitting. Their work, recently published in Nature Energy, demonstrates that graphite-protected bulk heterojunction organic photoactive layers can achieve unprecedented photocurrent densities alongside unparalleled operational stability in photoelectrochemical (PEC) cells. This remarkable development not only brings organic semiconductor-based devices to the forefront of solar fuel technology but also challenges the dominance of traditional inorganic materials, offering a sustainable and efficient path toward solar-driven hydrogen production.
At the heart of solar water splitting lies the ability of photoanodes to efficiently oxidize water molecules by harnessing sunlight, generating oxygen and protons which then recombine on the cathode side to form hydrogen fuel. Historically, this process has relied heavily on inorganic semiconductors such as silicon, hematite, and metal oxides. While these materials deliver respectable performance, they often suffer from scarcity, toxicity concerns, and costly fabrication methods. Organic semiconductors, by contrast, present a tantalizing alternative: earth-abundant, lightweight, and potentially low-cost with tunable optoelectronic properties. Yet, their application in water oxidation has been hampered by modest photocurrent densities and poor operational lifetimes, often restricted to mere minutes or hours of active use.
The new study makes a decisive leap by demonstrating organic IPV-anodes (integrated photoanode-photoabsorber with semiconductor interlayers) that break the 25 mA/cm² photocurrent density ceiling, registering values as high as 26.4 mA/cm² for PM6:D18:L8-BO devices and 23.7 mA/cm² for PM6:PY-IT devices at +1.23 V_RHE. These figures substantially surpass previous benchmarks for organic semiconductor-based devices, where photocurrents rarely exceeded 5 mA/cm², save for a solitary prior report peaking at 15 mA/cm². Such a leap is indicative of both superior light absorption and charge separation efficiencies, signaling that organic materials can now rival—and, in some scenarios, exceed—the performance of conventional inorganic counterparts.
Integral to this advancement is the strategic incorporation of graphite protection layers overlaying the bulk heterojunction active layers. This graphite shield dramatically enhances chemical robustness against the harsh aqueous environments typical in PEC water oxidation, effectively mitigating degradation pathways that have long plagued organic photoelectrodes. Consequently, these devices demonstrate operational stability extending over multiple days, a stark contrast to the limited durability exhibited by earlier organic photoanodes, which were prone to rapid performance decay within minutes or hours. The extended lifetime is a crucial milestone, underscoring the potential for real-world application and scalability.
Beyond raw photocurrent density and stability, the paper delves into the nuanced relationship between the active layer’s bandgap and the photoelectrochemical onset potential (E_on), critical parameters for maximizing solar-to-hydrogen conversion efficiency. The PM6:D18:L8-BO organic IPV-anode notably balances a favorable bandgap—tailored to efficiently absorb visible sunlight—and a low onset potential, facilitating an early rise in photocurrent with applied bias. Compared with other IPV-anodes employing inorganic semiconductors like perovskites or silicon, this organic counterpart achieves near-ideal performance relative to its bandgap, positioning it close to the Shockley-Queisser theoretical limit for photocurrent density.
While silicon-based IPV-anodes continue to lead in absolute photocurrent produced, these devices operate with notably higher onset potentials (+0.9 V_RHE or greater), partly due to silicon’s narrower 1.1 eV bandgap. Such higher onset potentials translate into increased external energy requirements for water oxidation initiation, potentially reducing overall efficiency. The organic devices presented strike a more compelling balance, delivering high photocurrents at comparatively lower onset potentials, and thus hold promise for more energy-efficient PEC systems.
This work also underscores a shift in the design philosophy of PEC devices. Traditional strategies have often centered on optimizing photoelectrode materials individually, frequently relying on sacrificial agents or complex multi-junction configurations to achieve enhanced performance. The fully integrated single-junction organic devices presented here operate without sacrificial reagents, offering a direct and practical pathway to unassisted water splitting. Such simplicity not only streamlines device architecture but also accelerates the translation from laboratory proof-of-concept to scalable, deployable solar fuel generators.
The implications of this breakthrough extend beyond incremental efficiency improvements. Organic photoactive layers such as those explored herein bring forth the possibility of flexible, lightweight, and cost-effective PEC devices amenable to large-area fabrication. The compatibility of these polymers with roll-to-roll printing and other scalable manufacturing techniques holds promise for democratizing access to solar fuel technologies, especially in regions where resource constraints limit the adoption of traditional semiconductor-based systems.
Moreover, the graphite protection strategy invites further exploration into hybrid architectures combining organic semiconductors with protective, conductive overlays to extend device lifetimes without compromising performance. This modular design approach could inspire innovations across related fields such as photovoltaics, photocatalysis, and even bioelectronic interfaces.
This study’s meticulous benchmarking against a broad spectrum of state-of-the-art devices—including traditional photoanodes, advanced IPV-anodes, and various inorganic photoabsorbers—provides indispensable perspectives on the trajectory of solar water splitting research. By compiling performance metrics like photocurrent density and onset potential within comprehensive comparison frameworks, the authors chart a clear roadmap for future material and device optimization.
In conclusion, the advent of organic IPV-anodes surpassing 25 mA/cm² photocurrent densities and exhibiting days-long operational stability heralds a transformative moment for solar-driven water oxidation technologies. This leap not only expands the utility of organic semiconductor materials into hitherto inaccessible realms of solar fuel production but also offers a compelling blueprint for combining high performance with durability and scalability. As the global community strives toward sustainable energy solutions, such innovations embody the synergy of materials science, electrochemistry, and device engineering that will power the clean energy technologies of tomorrow.
The path forward will undoubtedly involve marrying these organic systems with complimentary catalysts, optimizing layer architectures for charge transport, and integrating smart encapsulation methods to push stability beyond current benchmarks. Additionally, real-world deployment trials and life-cycle assessments will be instrumental to fully ascertain the environmental and economic impacts of these novel PEC devices. Nonetheless, the foundation laid by this study is unequivocally strong, promising a future where sunlight not only generates electricity but also sustainably drives the production of clean hydrogen fuel through the prism of organic material innovation.
This research opens an inspiring new chapter in the quest for efficient, stable, and scalable solar water splitting platforms, breaking the boundaries between organic and inorganic systems, and setting a new standard in artificial photosynthesis.
Subject of Research: Organic photoactive layers for solar water oxidation and unassisted water splitting in PEC devices.
Article Title: Enhanced solar water oxidation and unassisted water splitting using graphite-protected bulk heterojunction organic photoactive layers.
Article References:
Daboczi, M., Eisner, F., Luke, J. et al. Enhanced solar water oxidation and unassisted water splitting using graphite-protected bulk heterojunction organic photoactive layers. Nat Energy (2025). https://doi.org/10.1038/s41560-025-01736-6
Image Credits: AI Generated
Tags: challenges of inorganic semiconductorsearth-abundant materials for energygraphite-protected semiconductorsoperational stability in solar devicesorganic photoactive layersorganic semiconductors in energy applicationsphotocurrent density improvementsphotoelectrochemical cellsrenewable energy advancementssolar fuel generationsolar water splitting technologysustainable hydrogen production
What's Your Reaction?
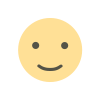
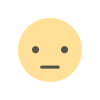
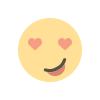
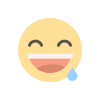
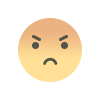
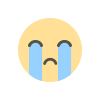
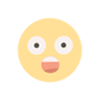