Engineering Breakthrough: Crafting the First Semimetallic Weyl Quantum Crystal
An international team of researchers from RIKEN Center for Emergent Matter Science (CEMS) has made history by successfully synthesizing an ideal Weyl semimetal, addressing a critical challenge that has persisted in the field of quantum materials for a decade. This groundbreaking achievement underscores the collective effort and ingenuity inherent within a collaborative research environment. Weyl […]

An international team of researchers from RIKEN Center for Emergent Matter Science (CEMS) has made history by successfully synthesizing an ideal Weyl semimetal, addressing a critical challenge that has persisted in the field of quantum materials for a decade. This groundbreaking achievement underscores the collective effort and ingenuity inherent within a collaborative research environment. Weyl fermions, emerging from the collective excitations of electrons in crystalline structures, are predicted to possess extraordinary electromagnetic properties that could lead to remarkable technological advancements.
Despite extensive research on a multitude of crystalline materials, most Weyl materials hitherto discovered have been overwhelmed by the influence of trivial electrons that obscure the presence of Weyl fermions. The successful synthesis of a material that supports a single pair of Weyl fermions without the interference of irrelevant electronic states represents not only a significant scientific breakthrough but also a culmination of years of theoretical predictions and experimental endeavors.
The research, published in the esteemed journal Nature, is the result of a four-year collaborative effort involving CEMS, the RIKEN Interdisciplinary Theoretical and Mathematical Sciences Program (iTHEMS), the Quantum-Phase Electronics Center (QPEC) at the University of Tokyo, the Institute for Materials Research at Tohoku University, and Nanyang Technological University in Singapore. The team ingeniously transformed a topological semiconductor into a Weyl semimetal, revisiting a strategy that had been theorized in 2011 but subsequently fell into relative obscurity within the scientific community.
Topological semiconductors, characterized by a small energy gap, can transition between insulating and conducting states. On the other hand, semimetals exist at the very brink of this transition, possessing a unique zero energy gap. This characteristic is exceedingly rare in natural materials, with graphene often cited as a prime example of a material featuring similar properties, particularly regarding its applications in flexible electronics and moiré physics.
The core material used in this groundbreaking study is bismuth telluride (Bi2Te3), a well-known topological semiconductor. Researchers carefully manipulated the chemical composition of the material by substituting chromium for bismuth, creating a compound denoted as (Cr,Bi)2Te3. This meticulous adjustment of the material’s properties allowed the team to unravel new physics beyond previously established topological semiconductor behavior, as evidenced by the observation of a large anomalous Hall effect (AHE).
The AHE observed in (Cr,Bi)2Te3 is particularly noteworthy, as it enables researchers to delve deeper into the material’s electronic structure. This uniquely simple electronic configuration has empowered the research team to quantitatively correlate their experimental results with theoretical predictions, thereby establishing a clear link between the large AHE and the emergent Weyl fermions. This connection signifies a pivotal moment in understanding quantum materials and their potential applications.
Leading author Ilya Belopolski expressed surprise at the discovery, noting that different research communities had already developed the necessary theoretical and experimental knowledge to synthesize this Weyl semimetal but had not effectively communicated. The success of this research illustrates the importance of collaboration across disciplines and highlights how missed opportunities can arise in the absence of dialogue between different scientific fields.
Belopolski attributed the emergence of this critical insight to the unique atmosphere fostered at RIKEN, where brilliant researchers come together in a creatively stimulating environment. The collaboration between talented research groups from various countries exemplifies the global pursuit of scientific knowledge and underlines how a collaborative approach can lead to significant breakthroughs that might otherwise remain unrealized.
One of the most exciting potential applications of this newly discovered Weyl semimetal lies in terahertz (THz) technology. Classical semiconductors are generally unable to absorb photons below certain energy thresholds dictated by their energy gaps. However, semimetals, with their zero energy gap, can effectively absorb light across the THz frequency range. This unique property positions Weyl semimetals as promising candidates for creating and detecting THz light, opening doors to potential advancements in communication technologies and sensor applications.
The implications of this discovery extend beyond just terahertz applications, as the research team anticipates exploration into high-performance sensors, low-power electronics, and innovative optoelectronic devices. The enthusiasm surrounding the prospects of this new quantum phase of matter embodies the dynamic research atmosphere at CEMS, where emerging technologies continuously push the boundaries of material science.
Lixuan Tai, a postdoctoral researcher who joined the Strong Correlation Quantum Transport Laboratory close to the publication of the findings, expressed exhilaration regarding the opportunities that this new Weyl semimetal presents for ongoing and future research. The team is poised to leverage the characteristics of this material to further explore its unique phases and properties, potentially sparking a wave of discoveries in quantum materials.
As researchers continue to delve into the properties of the ideal Weyl semimetal, they anticipate a rich landscape of inquiry that will lead to new methodologies and technological innovations. The intersection of theory and experimentation in this context illustrates the remarkable progress being made in the understanding of quantum materials, a field that will undoubtedly yield significant advancements in science and technology for years to come.
The synthesis of the ideal Weyl semimetal thus represents a transformative achievement in the realm of quantum transport and materials science. It paves the way for further exploration and understanding of Weyl fermions and their associated electromagnetic properties, signifying a potential turning point in how researchers approach the study of quantum materials and highlights the value of collaboration in unlocking the mysteries of the universe.
As the research community continues to build upon this foundation, the exciting prospects for the development of new devices, sensors, and methodologies driven by the unique properties of this Weyl semimetal will likely be a central theme in future scientific discourse. This breakthrough not only illustrates the potential of quantum materials but also serves as an exemplar of what can be achieved through sustained collaboration and innovative thinking in scientific research.
Subject of Research: Quantum Materials
Article Title: Synthesis of a semimetallic Weyl ferromagnet with point Fermi surface
News Publication Date: 22-Jan-2025
Web References:
References:
Image Credits:
Keywords
Tags: collaborative scientific breakthroughscrystalline structures and electronselectromagnetic properties of materialsinterdisciplinary research in materials scienceinternational research collaborationsNature journal publicationquantum materials researchRIKEN Center for Emergent Matter Sciencetechnological advancements in quantum physicstheoretical predictions in physicsWeyl fermions propertiesWeyl semimetal synthesis
What's Your Reaction?
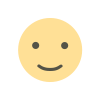
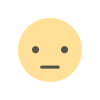
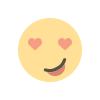
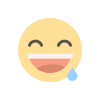
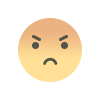
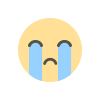
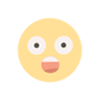