Capturing Vibrational Sum-Frequency Signals from Molecules Trapped in Nanoscale Gaps via Tightly Confined Optical Near-Fields
In a groundbreaking advance that promises to revolutionize molecular spectroscopy and nanoscale surface analysis, researchers have unveiled a novel technique that pushes the limits of spatial resolution far beyond conventional capabilities. Utilizing a cutting-edge tip-enhanced vibrational sum-frequency generation spectroscopy (TE-VSFG) system based on scanning tunneling microscopy (STM), scientists have achieved the direct detection of vibrational […]

In a groundbreaking advance that promises to revolutionize molecular spectroscopy and nanoscale surface analysis, researchers have unveiled a novel technique that pushes the limits of spatial resolution far beyond conventional capabilities. Utilizing a cutting-edge tip-enhanced vibrational sum-frequency generation spectroscopy (TE-VSFG) system based on scanning tunneling microscopy (STM), scientists have achieved the direct detection of vibrational signals from just a handful of molecules within an incredibly confined nanoscale junction. This pioneering work unlocks new avenues for exploring molecular orientation, ultrafast dynamics, and chemical processes at surfaces with unsurpassed precision and sensitivity.
Vibrational sum-frequency generation (VSFG) spectroscopy has long stood as a cornerstone technique for probing molecular structures at interfaces, owing to its intrinsic surface specificity and sensitivity to molecular vibrations. By simultaneously illuminating a sample with pulsed infrared and near-infrared lasers, VSFG generates a signal at the sum frequency, which encodes molecular vibrational fingerprints unique to species at surfaces or interfaces. These signals provide invaluable insights into molecular conformation, bonding, and orientation, all critical parameters across fields from catalysis to materials science.
However, traditional VSFG spectroscopy operates within the constraints of far-field diffraction limits, where spatial resolution struggles to break below sub-micrometer scales. This fundamental limitation means that the acquired spectra often represent averaged information from ensembles composed of millions or more molecules, obscuring vital nanoscale heterogeneities and localized behaviors. The inability to isolate molecular signals from nanometer-sized domains has posed a formidable challenge, particularly when studying complex, inhomogeneous, or dynamic interfaces where molecular-scale resolution is essential.
By integrating VSFG with scanning tunneling microscopy, the team led by Atsunori Sakurai and collaborators from the Institute for Molecular Science, NINS, have ingeniously circumvented this hurdle. The STM tip acts as a nanometric antenna, concentrating femtosecond pulsed mid-IR and near-IR laser radiation within the sub-nanometer gap between the tip and the gold substrate. This configuration generates an intense, highly confined optical near-field, which enhances the VSFG signal emanating exclusively from the few molecules residing inside the nanogap region.
Experimental data compellingly demonstrate the efficacy of this tip-enhanced system. When the STM tip is positioned at a distance exceeding 50 nanometers from the substrate, the VSFG signal becomes negligible. As the tip approaches into the tunneling regime—distances on the order of a nanometer—the VSFG intensity surges dramatically, unequivocally indicating the near-field enhancement effect. This spatial confinement was precisely controlled, revealing that detectable signals vanish when the tip-sample gap surpasses roughly one nanometer. Such sharp distance dependence affirms that the observed response originates strictly from the nanoscale junction.
Delving deeper into the spectral characteristics, the team meticulously tuned the mid-infrared excitation wavelength and recorded multiple VSFG spectra revealing distinct vibrational modes of the terminal methyl groups in the adsorbed molecules. These include symmetric stretching vibrations, a Fermi resonance involving bending overtone and symmetric stretching, and asymmetric stretching modes. Crucially, interferometric phase analysis of the spectral data allowed for extraction of the imaginary component of the vibrational resonant second-order susceptibility, Im[χ_R^(2)(ω_IR)], providing direct insights into molecular orientation.
This phase-sensitive detection disclosed negative values of Im[χ_R^(2)(ω_IR)], signifying that the terminal methyl groups orient their hydrogen atoms pointing away from the gold substrate surface. This finding underscores the technique’s remarkable capability to not only detect vibrational signatures from minute molecular populations but also to determine their absolute orientation with nanoscale spatial resolution. Such precise orientation mapping is vital in understanding surface reactivity, molecular self-assembly, and interfacial phenomena that dictate chemical function.
The profound near-field enhancement enabling this sensitivity emerges from two synergistic mechanisms. First, the antenna effect of the STM metallic tip effectively funnels and concentrates the mid-infrared electromagnetic fields onto the tip apex. Second, plasmonic enhancement arises within the nanogap formed between the metallic tip and substrate, boosting the efficiency of sum-frequency signal emission in the visible spectrum. These combined effects amplify electromagnetic fields to unprecedented strengths, dramatically elevating the signal-to-noise ratio and enabling the interrogation of sparse molecular populations.
Such nanoscale confinement and enhancement of the VSFG process represent a transformative milestone. The ability to detect signals from molecules restricted within less than one nanometer across a region consisting of only a few tens to hundreds of molecules opens the prospect of pushing this approach towards single-molecule detection. Moreover, ultrafast femtosecond pulse excitation incorporated in the setup positions this technique as a powerful tool to track ultrafast nuclear and electronic dynamics at molecular interfaces in real time.
From an application standpoint, the ramifications are far-reaching. Surface chemical reactions, heterogeneous catalysis, molecular electronics, and energy conversion processes often hinge on localized molecular behaviors inaccessible by ensemble-averaged measurements. TE-VSFG spectroscopy offers a window into these nanoscale processes, furnishing chemical, structural, and dynamical information with molecular specificity. Insights gained may facilitate the tailored design of catalysts with enhanced activity and selectivity or advance molecular-scale devices with refined control over interfacial properties.
Beyond practical applications, this research represents a significant conceptual leap bridging nonlinear optical spectroscopy and nanoscale surface science. It establishes a versatile platform where vibrational nanospectroscopy is performed under ambient conditions without requiring ultrahigh vacuum or cryogenic setups, broadening accessibility to diverse experimental environments. Combining precise tip positioning with phase-sensitive vibrational spectroscopy within an ultrafast pulsed laser framework heralds new frontiers in molecular-scale characterization.
In summary, the development of tip-enhanced vibrational sum-frequency generation spectroscopy grounded in scanning tunneling microscopy embodies a groundbreaking advance in nanoscale molecular spectroscopy. By harnessing intense near-field enhancements within sub-nanometer junctions, this technique captures molecular vibrational fingerprints from an unprecedentedly small number of molecules and reveals their orientation at surfaces with exquisite spatial precision. Expected to catalyze transformative progress in chemical physics, surface science, and nanotechnology, TE-VSFG offers a powerful lens into the hidden nanoscale world of molecules.
As this approach continues to mature, future directions may include extending spectral range, integrating complementary scanning probe methods, and pushing sensitivities towards the single-molecule threshold. This exciting pathway opens vast prospects for unraveling molecular transformations and dynamics at interfaces with chemical and spatial resolution previously unimaginable. The synergy of nonlinear optics, plasmonics, and scanning probe microscopy embodied here stands poised to rewrite the rules of vibrational nanospectroscopy and molecular imaging.
Subject of Research: Not applicable
Article Title: Tip-Enhanced Sum Frequency Generation for Molecular Vibrational Nanospectroscopy
News Publication Date: 10-Apr-2025
Web References: DOI: 10.1021/acs.nanolett.4c06065
Image Credits: Atsunori Sakurai
Keywords
Tip-enhanced spectroscopy, vibrational sum-frequency generation, scanning tunneling microscopy, nanospectroscopy, molecular orientation, plasmonic enhancement, near-field optics, ultrafast spectroscopy, surface chemistry, molecular vibrations, nonlinear optics, nanoscale characterization
Tags: advanced optical near-field techniqueschemical bonding analysis using VSFGhigh-resolution spectroscopy advancementsmolecular orientation measurement methodsnanoscale molecular spectroscopyprecision measurement in materials scienceresolving molecular structures at interfacesscanning tunneling microscopy applicationssurface analysis at nanoscaletip-enhanced vibrational sum-frequency generationultrafast dynamics in chemical processesvibrational signals detection techniques
What's Your Reaction?
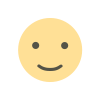
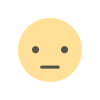
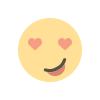
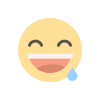
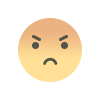
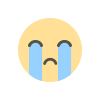
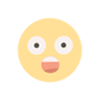