Can DNA Nanoparticle Motors Match the Speed of Motor Proteins?
In the realm of scientific innovation, few areas have garnered as much interest and potential as the development of artificial molecular motors. Among the most promising advancements are DNA-nanoparticle motors, which are intricate creations that harness the natural properties of DNA and RNA to facilitate motion. These tiny marvels operate through a mechanism known as […]

In the realm of scientific innovation, few areas have garnered as much interest and potential as the development of artificial molecular motors. Among the most promising advancements are DNA-nanoparticle motors, which are intricate creations that harness the natural properties of DNA and RNA to facilitate motion. These tiny marvels operate through a mechanism known as enzymatic RNA degradation, effectively converting chemical energy into mechanical movement. At the heart of their operation is the Brownian ratchet mechanism, which allows these motors to exploit the inherent randomness of particle motion, thereby promoting forward movement through the degradation of bonds along their path.
Despite the remarkable ingenuity behind DNA-nanoparticle motors, researchers have identified a significant limitation: their velocity pales in comparison to that of natural motor proteins found within living organisms. This discrepancy poses a challenge in leveraging the full potential of artificial motors in various applications, from drug delivery systems to molecular computations. Thus, the scientific community has mobilized efforts to analyze, optimize, and enhance the performance of these artificial motors, aspiring to close the speed gap that currently exists between synthetic constructs and their biological counterparts.
The primary focus of ongoing research has been elucidating the mechanisms that impede the speed of DNA-nanoparticle motors. In a breakthrough study published in the esteemed journal Nature Communications, a team of scientists investigated the factors contributing to this speed limitation, with a keen eye on the enzymatic interactions that play a pivotal role in the motor’s operational efficiency. Led by Takanori Harashima, the researchers employed single-particle tracking experiments alongside geometry-based kinetic simulations to uncover critical insights that could inform the engineering of faster motors.
Natural motor proteins are distinguished by their impressive speeds, typically ranging from 10 to 1000 nanometers per second. Conversely, traditional designs of artificial molecular motors have struggled to achieve such velocities, with most designs languishing under the 1 nanometer per second threshold. This stark contrast underscores the urgency to innovate and explore alternative solutions for enhancing the functionality of DNA-nanoparticle motors. The researchers, driven by the desire to push the boundaries of artificial motor technology, sought to identify and address specific bottlenecks that hindered performance.
Their investigation revealed that the enzyme RNase H was the primary bottleneck affecting motor speed. This enzyme plays an essential role in genome maintenance by breaking down RNA within RNA/DNA hybrids. The researchers ascertained that the efficiency of RNase H binding directly correlated with the overall processing time of the motor. A slower binding rate resulted in prolonged pauses during movement, significantly impeding the motor’s ability to generate rapid and consistent motion. Remarkably, by increasing the concentration of RNase H, the team observed a dramatic reduction in pause lengths, transitioning from an average of 70 seconds to a mere 0.2 seconds, thereby enhancing motor speed considerably.
However, this pursuit of speed was not without its trade-offs. As the researchers increased the speed of the DNA-nanoparticle motor, they noted a corresponding decline in two critical parameters: processivity and run-length. Processivity refers to the number of productive steps a motor can take before detaching from its substrate, while run-length denotes the distance it travels before losing attachment. The team discovered that by optimizing the hybridization rate between DNA and RNA, they could address these trade-offs and improve the overall performance of the motor.
Through meticulous engineering of DNA and RNA sequences, the researchers achieved a remarkable breakthrough. They presented a new configuration of the DNA-nanoparticle motor, which not only reached a speed of 30 nanometers per second but also exhibited processivity levels reaching 200 and a run-length of 3 micrometers. This astonishing performance showcased the potential for DNA-nanoparticle motors to rival their biological counterparts, signaling a new era in the development of artificial molecular motors.
The implications of this research extend beyond mere speed enhancements. The engineered DNA-nanoparticle motors hold promise for a myriad of applications, ranging from advanced molecular computation devices to highly sensitive diagnostic tools capable of identifying infectious agents and disease-associated molecules. By replicating and even surpassing the efficiency of natural motor proteins, these artificial motors could revolutionize the field of nanotechnology, paving the way for unprecedented advancements in various scientific domains.
As interest in the field of molecular motors continues to surge, researchers are motivated by an ambitious vision: to engineer artificial motors that not only match but exceed the capabilities of natural motor proteins. Such advancements could yield significant breakthroughs in medical diagnostics, therapeutic interventions, and fundamental biological research. The researchers behind this study believe that their findings serve as a stepping stone toward this transformative goal, providing insights that future studies may build upon to further discriminate and enhance motor performance.
The research carried out by Harashima and his colleagues at the Institute for Molecular Science and associated institutions is a testament to the collaborative efforts of scientists striving to push the frontiers of nanotechnology. With the support of various funding bodies, including JSPS KAKENHI and the Tsugawa Foundation, the researchers have laid the groundwork for future innovations in the realm of DNA-nanoparticle motors and artificial molecular machines.
The journey toward optimal artificial motors continues, driven by relentless curiosity and scientific inquiry. As researchers delve deeper into the intricacies of molecular motion and enzymatic interactions, the prospect of creating machines that can rival nature becomes increasingly feasible. The findings of this latest study not only advance our understanding of DNA-nanoparticle motors but also ignite excitement for what lies ahead in this captivating frontier of science.
In summary, the ongoing exploration into DNA-nanoparticle motors unveils a complex interplay of speed, efficiency, and utility, highlighting both the challenges and potential of these cutting-edge inventions. The race to engineer artificial molecular motors that mirror, and perhaps transcend, the capabilities of natural systems is far from over. Instead, it is an exhilarating journey that holds boundless possibilities for future innovations in nanotechnology, biomedicine, and beyond.
Subject of Research: Engineering of DNA-nanoparticle motors
Article Title: Rational engineering of DNA-nanoparticle motor with high speed and processivity comparable to motor proteins
News Publication Date: January 16, 2025
Web References: Nature Communications
References: Takanori Harashima, Akihiro Otomo, Ryota Iino
Image Credits: Illustration by Takanori Harashima
Keywords
DNA-nanoparticle motors, artificial molecular motors, motor proteins, enzymatic RNA degradation, nanotechnology, molecular computation, diagnostics, RNase H, hybridization rate, processivity, run-length, Nature Communications.
Tags: artificial molecular motorsdiagnosticsDNA-nanoparticle motorsenzymatic RNA degradationhybridization ratemolecular computationmotor proteinsnanotechnologyNature Communications.processivityRNase Hrun-length
What's Your Reaction?
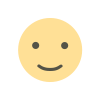
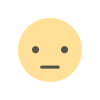
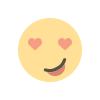
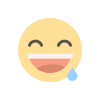
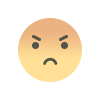
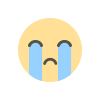
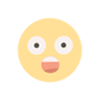