Breaking New Ground: Uncovering the Anomalous Hall Effect Without Magnetization in Novel Materials
In a breakthrough that challenges long-standing conventions in condensed matter physics, an international collaboration of scientists has unveiled the presence of the anomalous Hall effect within a collinear antiferromagnet, despite the absence of net magnetization. This discovery, led by researchers from the University of Tokyo and Johns Hopkins University, reveals an unexpected manifestation of electronic […]

In a breakthrough that challenges long-standing conventions in condensed matter physics, an international collaboration of scientists has unveiled the presence of the anomalous Hall effect within a collinear antiferromagnet, despite the absence of net magnetization. This discovery, led by researchers from the University of Tokyo and Johns Hopkins University, reveals an unexpected manifestation of electronic transport phenomena emerging from a non-Fermi liquid state, marking a transformative step in our understanding of magnetic materials and their applications in future information technologies.
For decades, the anomalous Hall effect has been closely associated with ferromagnets, where aligned electron spins generate a spontaneous magnetization that deflects electrical currents transverse to the applied electric field, giving rise to a Hall voltage without an external magnetic field. Ferromagnets’ uniformly aligned spins create robust internal magnetic fields fundamentally driving this phenomenon. Conversely, in antiferromagnets, the antiparallel alignment of spins cancels out net magnetization, rendering the anomalous Hall effect seemingly improbable. Yet, this emerging research shatters that notion by detecting a pronounced anomalous Hall effect in a material class previously considered incompatible with such behavior.
The material at the heart of this discovery is a specially engineered version of the layered compound V(_{1/3})NbS(_2), a transition metal dichalcogenide (TMD), which offers a versatile platform due to its layered, quasi-two-dimensional architecture. By intercalating magnetic vanadium ions between layers, researchers have effectively transformed the system into a three-dimensional structure with intricately tunable electron interactions and magnetic order. This fine control over the atomic-scale arrangement permits exploration of emergent quantum behaviors unattainable in conventional two-dimensional materials, ultimately enabling the observation of the anomalous Hall effect across various temperatures and magnetic field strengths.
Underlying this remarkable phenomenon is an intricate interplay between band topology—a concept describing the global geometrical properties of electronic energy bands—and strong electron correlations characteristic of non-Fermi liquid systems. Unlike classical Fermi liquids, where electrons behave as long-lived quasiparticles, non-Fermi liquids exhibit anomalous scattering and relaxation processes that defy simple particle descriptions. In V(_{1/3})NbS(_2), these interactions give rise to an unusual electronic environment that apparently generates a substantial “virtual magnetic field,” influencing charge carriers in a manner that mimics the effect of intrinsic magnetization, despite the true magnetic moments cancelling out at the macroscopic scale.
Advanced experimental techniques were paramount to this discovery. The team employed sensitive electrical transport measurements on carefully synthesized samples to isolate the Hall signal originating purely from the intrinsic electronic structure, disentangling it from conventional magnetic contributions that had obscured earlier observations in similar materials. Complementary neutron scattering experiments provided definitive microscopic confirmation of the collinear antiferromagnetic spin alignment, validating the absence of net magnetization and thereby affirming the unconventional origin of the observed Hall effect.
Theoretical insights were equally crucial in constructing a comprehensive framework to interpret these puzzling results. Computational analyses led by collaborators at the University of Tokyo utilized state-of-the-art band structure calculations incorporating strong correlation effects to elucidate the topological properties responsible for generating the effective Berry curvature—a geometric phase accumulating in momentum space—which acts analogously to a magnetic field for charge carriers. This realization connects the experimental findings to a rapidly expanding frontier in condensed matter physics, linking magnetism, topology, and electron correlations in previously unexplored ways.
Unlike prior weaker signals reported in other collinear antiferromagnets, this study provides the first robust and reproducible evidence of a large anomalous Hall effect completely devoid of ferromagnetic magnetization, an achievement that overturns textbook assumptions and opens avenues for leveraging antiferromagnets in next-generation spintronic devices. Antiferromagnetic materials offer intrinsic advantages over ferromagnets, including ultrafast spin dynamics, robustness against external magnetic noise, and compatibility with miniaturized device architectures, underscoring the transformative technological potential of this discovery.
Despite the groundbreaking nature of these results, the research team acknowledges considerable challenges remain in fully unraveling the microscopic mechanisms and generalizing the phenomenon across material systems. Structural disorder inherent to transition metal dichalcogenide frameworks complicates the interpretation of experimental data, necessitating meticulous characterization and sophisticated modeling. To address these complexities, the team plans to employ additional spectroscopic methods such as Raman scattering, resonant X-ray spectroscopy, and muon spin rotation techniques, aiming to probe the subtle interplay between lattice vibrations, electronic states, and magnetic order.
This finding not only enriches the fundamental understanding of emergent phenomena in correlated electron systems but also invigorates the search for new quantum materials exhibiting exotic electronic responses unattainable through classical mechanisms. By bridging deep theoretical concepts with innovative experimental protocols, this research exemplifies how foundational science fuels practical innovation, potentially catalyzing the development of ultra-efficient, high-speed information technologies that exploit magnetic degrees of freedom without the drawbacks posed by traditional ferromagnetic components.
As the pursuit of unconventional quantum states accelerates, the notion that an entirely magnetization-free anomalous Hall effect can exist foreshadows a redefinition of the criteria by which magnetic materials are classified and harnessed. The anticipated follow-up studies promise to shed further light on the rich physics embedded in transition metal dichalcogenides, paving the way for targeted material design using topological and correlated phenomena to engineer bespoke electronic functionalities.
In essence, this discovery heralds a paradigm shift, demonstrating that antiferromagnets are far more complex and technologically versatile than previously thought. It establishes a new chapter in condensed matter physics where the emerging synergy between topological band structures and non-Fermi liquid behavior unlocks novel electronic properties, inviting a reassessment of magnetic effects from first principles and inspiring future generations of scientific inquiry and innovation.
Subject of Research: Not explicitly stated in the original text.
Article Title: Zero-field Hall effect emerging from a non-Fermi liquid in a collinear antiferromagnet V(_{1/3})NbS(_2)
News Publication Date: 18-Apr-2025
Web References: https://doi.org/10.1038/s41467-025-58476-0
References: Ray et al., Nature Communications, 2025
Image Credits: Ray et al., 2025
Keywords
Antiferromagnetism, anomalous Hall effect, non-Fermi liquid, transition metal dichalcogenides, collinear antiferromagnet, band topology, Berry curvature, spintronics, quantum materials, electron correlations, V(_{1/3})NbS(_2), magnetic ions intercalation
Tags: anomalous Hall effectAntiferromagnetic materialscollinear antiferromagnetcondensed matter physics breakthroughselectronic transport phenomenainformation technology applicationsJohns Hopkins University collaborationmagnetic materials researchnon-Fermi liquid statetransition metal dichalcogenidesunconventional magnetizationUniversity of Tokyo research
What's Your Reaction?
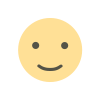
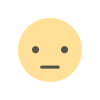
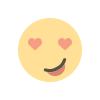
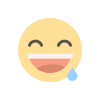
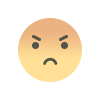
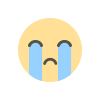
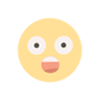