Boosting Stem Cell-Derived Islet Survival in Hypoxia
In recent years, the advent of stem cell technology has revolutionized regenerative medicine, offering hope for the treatment of chronic diseases that have long eluded definitive therapies. One such disease is diabetes mellitus, characterized by the dysfunction or loss of pancreatic islet cells responsible for insulin production. The ability to generate human stem cell-derived islets […]

In recent years, the advent of stem cell technology has revolutionized regenerative medicine, offering hope for the treatment of chronic diseases that have long eluded definitive therapies. One such disease is diabetes mellitus, characterized by the dysfunction or loss of pancreatic islet cells responsible for insulin production. The ability to generate human stem cell-derived islets holds enormous therapeutic potential, potentially circumventing the limitations associated with donor islet transplantation. However, a persistent challenge remains: ensuring these engineered islets can survive and function effectively when exposed to the harsh microenvironment often marked by low oxygen, or hypoxia. In a groundbreaking study published in Nature Communications, Wang et al. address this very issue, revealing novel strategies to improve the cellular fitness of stem cell-derived islets under hypoxic conditions, signaling a significant step forward in diabetes treatment and islet transplantation biology.
The profound difficulties that stem cell-derived islets face under hypoxia arise from the metabolic and physiological demands these cells bear during engraftment and long-term functionality. The native pancreatic islets in a healthy pancreas reside in a highly vascularized niche with adequate oxygen supply, which is crucial for their glucose sensing and insulin secretion functions. When transplanted, whether derived from donors or engineered from stem cells, islets often encounter an ischemic microenvironment wherein oxygen levels drop dramatically. This acute or chronic hypoxic stress leads to reactive oxygen species (ROS) accumulation, mitochondrial dysfunction, and ultimately cellular apoptosis or necrosis. Understanding and mitigating these damage pathways is essential to improving graft survival and therapeutic outcomes.
Wang and colleagues undertook a multifaceted approach grounded in cutting-edge cellular and molecular biology techniques to dissect the mechanisms by which human pluripotent stem cell-derived islets respond to hypoxia. Through systematic exposure of engineered islets to controlled oxygen deprivation, the researchers identified key signaling pathways disrupted under hypoxic stress, particularly those involving hypoxia-inducible factors (HIFs), metabolic reprogramming routes, and the unfolded protein response (UPR) associated with endoplasmic reticulum stress. They found that hypoxia triggers a cascade of intracellular events that impair insulin secretion, reduce cellular proliferation, and sensitize islets to apoptosis-inducing signals.
Crucially, the study proposed and validated interventions aimed at enhancing islet resilience to hypoxia. By modulating HIF signaling with small molecules and upregulating endogenous antioxidant defenses via genetic and pharmacologic means, the researchers could restore mitochondrial function and preserve cellular viability in vitro. These interventions not only maintained the energetic demands of the islets but also improved their insulin secretory response during hypoxia, a benchmark of functional integrity. Notably, these protective modifications did not compromise the differentiation status or cellular identity of the stem cell-derived islets, an important consideration for translational application.
The technical sophistication of this study is underscored by the application of single-cell transcriptomics and metabolic flux analyses, which allowed an unprecedented resolution into the heterogeneity of islet cell populations and their individual responses to oxygen deprivation. This granular insight underscored that not all islet subpopulations are equally vulnerable; beta cells, which produce insulin, demonstrated distinct vulnerability profiles compared to alpha and delta cells. These findings may refine future strategies that selectively bolster the robustness of the most critical endocrine cell types, yielding more efficacious islet constructs optimized for transplantation.
Another remarkable aspect of this work centers on the bioengineering advancements integrated into their experimental platform. The team employed 3D culture systems mimicking the native extracellular matrix of the pancreatic niche to study cell-cell and cell-matrix interactions under hypoxia. Such environments better reproduce in vivo conditions compared to traditional 2D cultures, thereby enhancing the clinical relevance of their findings. Additionally, the study introduced innovative oxygen-sensing biosensors within these cultures, enabling real-time monitoring of O2 gradients and cellular responses, a powerful tool for iterative refinement of islet engineering.
The implications of improving cellular fitness under hypoxia extend beyond transplantation survival to potentially enhance the scalability and manufacturing viability of stem cell-derived islet products. Longer-lasting and functionally competent islets could reduce the number required per patient, easing the bottleneck of cell production and facilitating broader clinical access. As the global diabetes burden escalates, with millions affected worldwide, such advancements may ultimately pivot stem cell-derived islet therapies from experimental interventions to mainstream clinical practice, transforming patient outcomes.
Importantly, this study also opens avenues for investigating hypoxia-associated stress responses in other stem cell-derived tissues and organoids, where oxygen supply similarly constrains therapeutic efficacy. The mechanisms elucidated and the bioengineering solutions proposed could act as templates or be adapted to improve survival in a myriad of regenerative medicine contexts. This cross-applicability enhances the broader significance of the work beyond islet biology alone.
Despite these promising findings, challenges remain, particularly concerning the translation of in vitro improvements to in vivo settings where the immune response, systemic metabolic demands, and complex microenvironmental factors further complicate islet survival and function. Nevertheless, the robust experimental design and multi-pronged strategies adopted in the study provide a compelling framework to address these hurdles incrementally.
Moreover, the study’s integration of genetic tools to promote endogenous protective pathways highlights a trend towards precision cellular engineering. By editing or modulating islet cells at the molecular level to intrinsically resist hypoxic injury, therapeutic products can be intrinsically more durable and less reliant on external adjuncts such as immunosuppression or oxygen-delivering scaffolds. This paradigm shift aligns well with personalized regenerative medicine, where tailored cell therapies adapt to the patient’s physiological and pathological milieu.
In addition to the biological and engineering aspects, Wang et al. critically examined metabolic dynamics within islets under hypoxic stress. Their metabolic flux assays demonstrated that hypoxia induces a switch from oxidative phosphorylation to glycolysis, a survival mechanism yet one that compromises energy efficiency and insulin secretion capacity. Therapeutic interventions that restore mitochondrial respiration without triggering detrimental oxidative stress thus represent a finely balanced strategy to enhance cellular fitness.
Another dimension explored in the study is the role of endoplasmic reticulum (ER) stress in hypoxia-related islet dysfunction. The accumulation of misfolded proteins under oxygen deprivation activates the unfolded protein response, which, if prolonged, leads to apoptosis. The researchers showed that pharmacologic modulation of UPR components can alleviate ER stress, preserving islet viability and secretory function during hypoxia. This finding expands the arsenal of therapeutic targets to improve islet resilience beyond metabolic or antioxidant pathways.
The clinical significance of this research cannot be overstated. Diabetes management currently depends heavily on exogenous insulin administration, which, while life-saving, fails to replicate physiologic glucose regulation and predisposes patients to complications. Stem cell-derived islets restored to robust health can revolutionize this landscape by offering a functional, physiologic insulin source, potentially curing or dramatically alleviating disease symptoms.
Looking ahead, the translational roadmap informed by this study includes optimization of islet culture and differentiation protocols to embed hypoxia resistance from inception, development of implantable devices facilitating vascularization and oxygen delivery, and eventual clinical trials assessing safety and efficacy in diabetic patients. Collaborative efforts among cell biologists, bioengineers, and clinicians will be crucial to realize the therapeutic promise demonstrated by Wang and colleagues.
In summary, the work published by Wang et al. in Nature Communications marks a pivotal advancement in regenerative medicine and diabetes therapy. By elucidating the molecular underpinnings of hypoxia-induced islet dysfunction and presenting viable interventions to enhance cellular fitness, this study paves the way for more effective and durable stem cell-derived islet transplantation. Such strides not only increase the feasibility of curing diabetes but also deepen our understanding of cellular adaptation and survival under adverse conditions, with broad biomedical implications.
Subject of Research: Improvement of cellular fitness and survivability in human stem cell-derived pancreatic islets under hypoxic conditions for enhanced transplantation outcomes.
Article Title: Improving cellular fitness of human stem cell-derived islets under hypoxia.
Article References:
Wang, X., Brielle, S., Kenty-Ryu, J. et al. Improving cellular fitness of human stem cell-derived islets under hypoxia. Nat Commun 16, 4787 (2025). https://doi.org/10.1038/s41467-025-59924-7
Image Credits: AI Generated
Tags: diabetes mellitus treatment advancementsenhancing islet engraftment successhypoxia challenges in islet transplantationimproving cellular fitness in engineered isletsmetabolic demands of stem cell-derived isletsNature Communications stem cell studynovel therapies for diabetes managementoxygen supply in pancreatic isletspancreatic islet cell dysfunctionstem cell technology in regenerative medicinestem cell-derived islets survival strategiesvascularization in tissue engineering
What's Your Reaction?
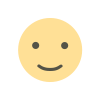
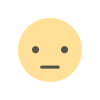
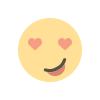
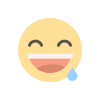
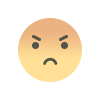
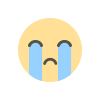
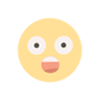