Boosting Optical Nonlinearities in Plasmonic Nanostructures
In a groundbreaking advancement that promises to redefine the future of photonics and optoelectronics, researchers have unveiled a novel method for controlling and enhancing optical nonlinearities within plasmonic semiconductor nanostructures. This pioneering work, led by Rossetti, Hu, Venanzi, and their colleagues, marks a significant leap in our understanding and manipulation of light–matter interactions at the […]

In a groundbreaking advancement that promises to redefine the future of photonics and optoelectronics, researchers have unveiled a novel method for controlling and enhancing optical nonlinearities within plasmonic semiconductor nanostructures. This pioneering work, led by Rossetti, Hu, Venanzi, and their colleagues, marks a significant leap in our understanding and manipulation of light–matter interactions at the nanoscale, with profound implications for the development of ultra-efficient optical devices and next-generation communication technologies.
Optical nonlinearities refer to phenomena where the response of a material to incident light is not linearly proportional to the light’s intensity. Such nonlinear effects are pivotal in enabling devices capable of light modulation, switching, frequency conversion, and ultrafast signal processing. However, harnessing strong optical nonlinearities typically requires high power or bulky components, which impede miniaturization and integration into compact systems. The approach of Rossetti and team leverages the unique properties of plasmonic semiconductor nanostructures to tailor and amplify these nonlinear effects effectively within a nanoscale footprint.
At the heart of this research are plasmonic resonances—collective oscillations of free electrons at the interface between a metal and a semiconductor, excited by incident light. These resonances create intensely localized electromagnetic fields, dramatically increasing light–matter interaction strength. By intricately designing semiconductor nanostructures that support plasmonic modes, the researchers have achieved unprecedented control over optical nonlinearities, allowing the tuning of these effects with remarkable precision.
Unlike traditional metallic plasmonics that often suffer from significant optical losses, the use of semiconductor materials offers distinct advantages. Semiconductors provide greater tunability through bandgap engineering and doping, facilitating optimization of plasmonic response and nonlinear behavior. Importantly, semiconductor plasmonics promise compatibility with existing electronic and photonic platforms, paving the way for seamless integration into current technology infrastructures.
The team’s innovative fabrication techniques enabled the creation of nanoscale architectures whose dimensions and compositions are meticulously controlled. These structures exhibit a strong interplay between plasmonic modes and intrinsic semiconductor nonlinearities, such as three-photon absorption and Kerr effects. Through careful experimental characterization complemented by theoretical modeling, the researchers demonstrated enhancement factors in nonlinear responses far exceeding those achievable in conventional materials or configurations.
One of the striking findings is the ability to dynamically modulate the intensity and phase of nonlinear optical signals by adjusting external parameters like excitation wavelength, intensity, and ambient environment. This flexible control is critical for practical applications, where device functionality must be adaptable to diverse and rapidly changing operational conditions. Moreover, by exploiting hot electron generation within plasmonic nanostructures, the team managed to access additional nonlinear pathways contributing to enhanced optical conversion efficiencies.
The implications for telecommunications are particularly exciting. The enhanced nonlinearities in these plasmonic semiconductor nanostructures enable ultrafast all-optical switching with energy footprints orders of magnitude lower than current technologies. This capability could lead to revolutionary improvements in data transmission rates, signal integrity, and network energy consumption, addressing key bottlenecks in the race toward faster and greener information infrastructure.
Beyond communications, the research holds promise for quantum photonics, where control over nonlinear interactions is essential for generating entangled photons and implementing quantum logic operations. The precise engineering of plasmonic resonances in semiconductor nanostructures may offer new pathways for scalable quantum devices operating at room temperature, overcoming limitations imposed by other materials systems.
The multidisciplinary approach combining materials science, nanofabrication, nonlinear optics, and theoretical physics underscores the collaborative spirit driving this cutting-edge development. Advanced computational simulations guided the design process, while state-of-the-art spectroscopy and microscopy techniques unveiled the complex dynamics underpinning observed phenomena. The synergy of theory and experiment enabled robust validation of results, enhancing confidence in their applicability.
Future directions highlighted by the authors include exploring different semiconductor compositions, integrating heterostructures, and incorporating external stimuli such as electric fields or temperature gradients to further modulate nonlinear responses. Such expansions could uncover new regimes of controllable optical phenomena, broadening the palette of functionalities accessible through plasmonic nanostructures.
This research also raises intriguing fundamental questions about the limits of light confinement and nonlinear interaction enhancement. By pushing the boundaries of nanoscale optics, the work stimulates both applied innovation and deeper inquiry into light–matter interactions, inspiring the scientific community to rethink conventional paradigms.
In practical terms, the findings open avenues for miniaturized, on-chip nonlinear optical components capable of performing complex signal processing tasks with low latency and high energy efficiency. This could accelerate the deployment of integrated photonic circuits in consumer electronics, healthcare diagnostics, and environmental sensing technologies.
Moreover, the scalable nature of semiconductor fabrication methodologies aligns well with industry demands, suggesting feasible pathways for commercial translation. The compatibility with silicon-based platforms further enhances the prospects for widespread adoption, potentially transforming multiple technology sectors simultaneously.
In essence, this breakthrough epitomizes the power of nanoscale engineering to transcend traditional material limitations, yielding novel functionalities through intricate control of electromagnetic phenomena. As the interplay between optics and semiconductor technology deepens, the horizon for transformative photonic devices expands, promising a future where light-driven innovation reshapes daily life.
Rossetti and collaborators have thus provided not only a demonstration of enhanced nonlinear optical properties but also a versatile platform and design principles that could catalyze advances across scientific disciplines. The fusion of semiconductor plasmonics with nonlinear optics emerges as a fertile ground for discovery and application, embodying the dynamic interplay between fundamental science and technological progress.
This landmark study sets a new benchmark in the quest for highly efficient, tunable, and compact nonlinear optical systems, ushering in an era of photonic innovations that blend nanoscale precision with practical utility. As researchers continue to unravel the complexities of plasmonic semiconductor nanostructures, the transformative potential of these materials stands poised to unlock unprecedented capabilities in optical technology.
Subject of Research: Control and enhancement of optical nonlinearities in plasmonic semiconductor nanostructures
Article Title: Control and enhancement of optical nonlinearities in plasmonic semiconductor nanostructures
Article References:
Rossetti, A., Hu, H., Venanzi, T. et al. Control and enhancement of optical nonlinearities in plasmonic semiconductor nanostructures. Light Sci Appl 14, 192 (2025). https://doi.org/10.1038/s41377-025-01783-4
Image Credits: AI Generated
DOI: https://doi.org/10.1038/s41377-025-01783-4
Tags: advancements in ultrafast signal processing technologiescontrolling nonlinear optical effects at nanoscaleenhancing electromagnetic field localization in photenhancing light-matter interactions in nanostructuresimplications of plasmonic resonances in light modulationminiaturization of optical components using plasmonicsnovel approaches to frequency conversion in nanostructuresnovel methods in photonics and optoelectronicsoptical nonlinearities in plasmonic materialsplasmonic semiconductor nanostructures for optical devices
What's Your Reaction?
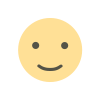
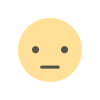
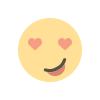
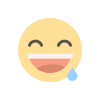
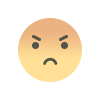
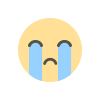
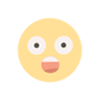