Antiferromagnetic Quantum Anomalous Hall Transformations
In the rapidly evolving field of topological materials, the discovery and exploration of intrinsic magnetic topological insulators have marked a profound milestone, especially with the advent of MnBi2Te4. This van der Waals layered antiferromagnet has emerged as a fertile platform that bridges the realms of magnetism and nontrivial band topology, offering unprecedented access to exotic […]

In the rapidly evolving field of topological materials, the discovery and exploration of intrinsic magnetic topological insulators have marked a profound milestone, especially with the advent of MnBi2Te4. This van der Waals layered antiferromagnet has emerged as a fertile platform that bridges the realms of magnetism and nontrivial band topology, offering unprecedented access to exotic quantum phases. The intrinsic interplay between its unique antiferromagnetic order and topological band structure has enchanted researchers aiming to unravel new physical phenomena that remain inaccessible in conventional ferromagnetic topological insulators.
Since its theoretical prediction and experimental realization, MnBi2Te4 has captivated intense scientific attention due to its ability to host the quantum anomalous Hall (QAH) effect coupled with antiferromagnetism. Traditionally, QAH states have been observed in ferromagnetic topological insulators, where spontaneous magnetization breaks time-reversal symmetry and induces chiral edge channels. The odd and even layering of MnBi2Te4, however, present fascinatingly different re-entries into QAH and axion insulator states respectively, underscoring the subtle influence of magnetic texture on topological edge modes.
Beyond static topological characterization, dynamic magnetism in MnBi2Te4 introduces an additional layer of complexity. The inherently antiferromagnetic spin configurations yield rich spin dynamics that have long been hypothesized to engender unconventional QAH phenomena. Unlike ferromagnetic systems where spin alignment is uniform, the layer-dependent spin flip and flop transitions characteristic of this van der Waals antiferromagnet create a multifaceted magnetic landscape that interacts intimately with the topological electronic states. This nuanced magneto-electronic coupling opens exciting avenues for quantum transport phenomena, awaiting experimental substantiation.
Addressing this experimental frontier, a recent breakthrough has been achieved by fabricating a device consisting of seven septuple layers of MnBi2Te4. This architecture is further stabilized by an aluminum oxide (AlOx) capping layer, which not only preserves the material’s intrinsic properties but also facilitates delicate control over its electronic landscape. Employing dual tuning knobs of gate voltage and external perpendicular magnetic field, researchers have conducted meticulous transport measurements, navigating through a dense parameter space that reveals a succession of quantum phase transitions. These transitions manifest as discrete changes in edge state conduction, directly linked to evolving spin textures within the layered antiferromagnet.
One of the most striking findings in this study lies in the response to in-plane magnetic fields, which contrast starkly with analogous ferromagnetic systems. Instead of suppressing or destabilizing the QAH state, the in-plane field in MnBi2Te4 enhances both the coercive field—the measure of magnetic hysteresis—and the exchange gap of the topological surface state. The exchange gap, crucial for robust topological protection, is a direct indicator of electronic band splitting driven by magnetic order. This anomalous enhancement underscores an intrinsic difference in how antiferromagnetic spin arrangements influence electronic states compared to ferromagnets.
The underlying mechanisms have been unraveled through comprehensive numerical simulations that align closely with experimental observations. These simulations portray how spin flip transitions—where the spin orientation abruptly reverses—and spin flop transitions—where spins reorient collectively in response to magnetic torque—modulate the macroscopic electronic topology of the system. Such transitions alter the edge channel connectivity and transport characteristics, underpinning the experimentally observed cascade of quantum phase transitions. This intricate dance between electron topology and spin dynamics typifies the quantum complexity possible in layered antiferromagnetic topological insulators.
Importantly, the tunability demonstrated in these MnBi2Te4 devices unlocks potential routes toward advanced spintronic applications. Unlike ferromagnetic counterparts, where spin manipulation often entails energetic penalties or limited control, antiferromagnetic topological insulators offer a robust platform for low-power, high-speed devices. Their topological states, sensitive to subtle spin reorientations, suggest new paradigms for information encoding and quantum state manipulation that leverage both charge and spin degrees of freedom.
In broader scientific context, this research positions MnBi2Te4 as a prime candidate for exploring axion electrodynamics and emergent phenomena rooted in symmetry-breaking topological phases. The coexistence of antiferromagnetism with nontrivial topology challenges classic theoretical frameworks and propels the development of novel models that integrate quantum magnetism with topological band theory. These findings are not simply an incremental advance but a leap toward a deeper understanding of quantum phases that could revolutionize both fundamental physics and technological innovation.
Moreover, these insights invigorate the pursuit of topological antiferromagnetic spintronics, a frontier promising practical devices capable of harnessing delicate quantum effects at ambient conditions. The delicate balance of spin flips and flops serving as control parameters offers compelling strategies for dynamic quantum state engineering, transcending the limitations faced by traditional ferromagnetic systems. Potential applications range from ultra-stable memory elements to dissipationless electronics and topologically protected quantum computation schemes.
Future research directions will likely focus on optimizing the material quality and device structures to enhance robustness and scalability. Combining multiple external tuning parameters, such as strain and electric fields, alongside magnetic fields, may further enrich the controllability over quantum phase landscapes. Furthermore, time-resolved spectroscopy and scanning probe techniques could provide direct visualization of spin rearrangements, illuminating the microscopic mechanisms that govern macroscopic transport phenomena.
The implications of this line of research also extend to other members of the MnBi2Te4 family and related van der Waals antiferromagnetic materials. Comparative studies might reveal universal principles or material-specific quirks that dictate the manifestation of QAH and axion insulating behaviors. Such understanding could facilitate material engineering efforts aimed at tailor-making quantum materials with desired topological and magnetic regimes.
In sum, the demonstration of antiferromagnetic quantum anomalous Hall effect modulated by spin flips and flops in MnBi2Te4 singularly advances the frontier of condensed matter physics. It exemplifies how intricate magnetic textures in novel quantum materials can yield unprecedented control over topological electronic states, paving the way toward revolutionary spintronic technologies rooted in fundamental quantum phenomena. As research continues to unfold, MnBi2Te4 stands as a beacon of the rich, untapped potential embedded within the interplay of magnetism and topology in low-dimensional materials.
Subject of Research: Antiferromagnetic quantum anomalous Hall effect and spin dynamics in MnBi2Te4 topological insulators
Article Title: Antiferromagnetic quantum anomalous Hall effect under spin flips and flops
Article References:
Lian, Z., Wang, Y., Wang, Y. et al. Antiferromagnetic quantum anomalous Hall effect under spin flips and flops. Nature (2025). https://doi.org/10.1038/s41586-025-08860-z
Image Credits: AI Generated
Tags: antiferromagnetic order and topologyantiferromagnetic quantum anomalous Hall effectdynamic magnetism and topological edge modesexotic quantum phases in condensed matterintrinsic magnetic materialsmagnetic texture in topological materialsMnBi2Te4 topological insulatornontrivial band topologyQAH states in antiferromagnetic systemsspin dynamics in antiferromagnetsunconventional quantum phenomenavan der Waals layered materials
What's Your Reaction?
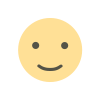
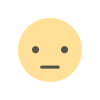
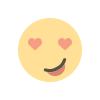
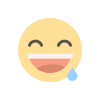
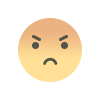
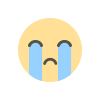
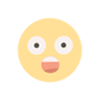