Acetate Metabolism Emerges as Critical Defender of Brain Function in Sleep-Disrupted States
In a world increasingly dominated by high-speed digital engagement and relentless pressure to optimize every waking moment, the fundamental importance of sleep is too often cast aside. Yet scientific research has repeatedly shown that the impact of sleep on health and overall well-being is both profound and indispensable. Sleep disturbances, in particular, are coming into […]

In a world increasingly dominated by high-speed digital engagement and relentless pressure to optimize every waking moment, the fundamental importance of sleep is too often cast aside. Yet scientific research has repeatedly shown that the impact of sleep on health and overall well-being is both profound and indispensable. Sleep disturbances, in particular, are coming into sharper focus as a global public health concern, as they have been implicated in a wide spectrum of metabolic, cognitive, cardiovascular, and immunological dysfunctions. Within this realm of disturbances, sleep fragmentation has emerged as a central culprit, posing significant risks to health by interrupting normal sleep architecture and consistently impairing the body’s ability to maintain homeostasis. Despite ongoing debates about ideal sleep duration and the precise function of different sleep stages, a growing consensus acknowledges that fragmented sleep patterns can lead to metabolic derangements, diminished cognitive performance, and a host of related dysfunctions. In the quest to uncover the molecular pathways that mediate these harmful outcomes, a novel set of discoveries about acetate, a short-chain fatty acid (SCFA), has begun to illuminate how the body may deploy a protective response in the face of chronic sleep disruption.
These new findings point to a reality that might sound paradoxical: while chronic sleep fragmentation triggers elevations in certain detrimental markers—ranging from impaired glucose metabolism to cognitive deficits—there also appears to be an adaptive mechanism that the hypothalamus exploits in order to mitigate some of the worst metabolic impacts of such disturbances. By examining a chronic sleep fragmentation mouse model, researchers discovered that the accumulation of acetate in the hypothalamus is not merely a by-product of dysregulated metabolism, but rather an adaptive response that can help safeguard the body from the full brunt of fragmented sleep’s deleterious effects. This discovery underscores the complexity of physiological responses to sleep disruption and opens new avenues for therapeutic interventions that could leverage gut microbiota-derived acetate to improve metabolic health and cognitive function.
In constructing a robust model to analyze these mechanisms, investigators selected adult C57BL/6J male mice—an established standard in biomedical research—and subjected them to two weeks of a carefully orchestrated chronic sleep fragmentation (SF) protocol. By placing an orbital rotor in operation during the light-ON phase, they were able to mimic the frequent disruptions to sleep that people experience in conditions like obstructive sleep apnea (OSA) or in certain age-related or neurodegenerative contexts. Their assessment of sleep architecture through electroencephalogram (EEG) and electromyography (EMG) confirmed that the SF mice indeed spent more time awake during the light period, demonstrated less non-rapid eye movement (NREM) sleep, and showed shifts in rapid eye movement (REM) sleep during the dark phase. While the total proportions of wake, NREM, and REM over a 24-hour period did not dramatically differ from controls, the SF mice showed a hallmark feature: more frequent transitions among these states, thus confirming a pattern of broken, less restorative sleep.
The consequences of this two-week period of sleep fragmentation proved striking. The SF mice exhibited impaired glucose metabolism, insulin resistance, and a range of metabolic alterations typically linked to disorders such as type 2 diabetes (T2D). Fasting plasma glucose levels were significantly elevated, and glucose tolerance tests (GTTs) revealed blunted glucose responses, pointing to a systemic issue in glucose handling. Insulin tolerance tests (ITTs) and calculations of homeostasis model assessment of insulin resistance (HOMA-IR) reinforced the conclusion that insulin signaling was impaired. In parallel, mice subjected to SF performed poorly in cognitive assessments such as the Morris water maze—used to evaluate spatial learning and memory—and the novel object recognition test, which measures recognition-based memory. Their difficulty in recalling the location of the hidden platform in the water maze or distinguishing new objects in a familiar setting demonstrated how SF affects not only the body’s metabolic and physiological functions but also critical cognitive processes.
To delve deeper into the specific molecular landscape associated with these impairments, researchers conducted plasma proteomic analyses comparing SF mice to controls. A number of proteins involved in glucose homeostasis were found to be significantly altered in the fragmented-sleep group, while 18F-fluorodeoxyglucose (FDG) positron emission tomography–computed tomography (PET-CT) scans showed diminished FDG uptake in key brain regions such as the hypothalamus. This result hinted at lower glucose utilization in areas that regulate metabolic and cognitive function. The use of [U-13C6] glucose tracing, an advanced technique enabling the tracking of labeled glucose through metabolic pathways, uncovered reduced incorporation of the 13C label in glycolytic and tricarboxylic acid (TCA) cycle intermediates within the hypothalamus, hippocampus, and cortex of SF mice. Collectively, these findings exposed how chronic sleep fragmentation perturbs insulin signaling and disrupts metabolic processes in the brain.
Intriguingly, these systemic and brain-centered metabolic disturbances were accompanied by comprehensive changes in the circulating metabolome, especially with regard to short-chain fatty acids. SCFAs such as acetate, propionate, and butyrate rose significantly in the plasma of mice experiencing chronic sleep fragmentation. Among them, acetate showed a unique, time-dependent increase over the course of the two-week disruption. A deeper look into the gut microbiota suggested an overabundance of certain genera capable of producing acetate, like Prevotellaceae, Dubosiella, and Desulfovibrio, while other bacterial genera associated with beneficial gut health, such as Akkermansia, Parasutterella, and Lachnospiraceae, diminished in number. This significant shift in microbial populations occurred alongside measurable damage to the intestinal epithelium, including decreased villous length and width and a marked downregulation of occludin, a tight junction protein critical for maintaining intestinal barrier integrity. Paradoxically, even though acetate-producing bacteria were more plentiful in the SF mice, the actual levels of acetate measured in the cecum and feces were lower than in controls, suggesting that increased gut permeability might have facilitated the leakage of acetate into the circulation rather than retaining it in the intestinal environment.
Further refinement of the data revealed that this acetate primarily accumulated in the hypothalamus of SF mice but not in other brain regions. A correlation emerged: the more frequent the transitions between NREM sleep and wakefulness, the higher the concentration of acetate detected both in the hypothalamus and in circulation. To follow acetate’s fate once it entered the brain, scientists used 13C-NMR isotopomer analysis in conjunction with [1,2-13C2] acetate labeling. Results indicated a reduction in acetate oxidation through the TCA cycle, accompanied by lower production of ATP. This partial blockade was associated with a decrease in the expression of acyl-coenzyme A synthetase short-chain family member (ACSS) 1 within the hypothalamus, indicating that less acetate was being converted into acetyl-CoA for energetic use in that specific region. Initially, one might surmise that this accumulation of acetate is detrimental, but experiments quickly demonstrated that it serves an adaptive, protective purpose.
Oral supplementation with acetate to mice subjected to chronic sleep fragmentation yielded multiple improvements: better glucose handling, enhanced insulin sensitivity, and significant gains in cognitive tests. These improvements were mirrored by reversing the detrimental impact of antibiotics that suppressed acetate production by gut bacteria. That is, when the gut microbiota was compromised and acetate levels fell, mice experienced a worsened metabolic profile and sharper cognitive deficits during sleep fragmentation, which then were restored by supplying exogenous acetate. Moreover, direct infusions of acetate into the central nervous system via intracerebroventricular delivery curtailed inflammation and improved metabolic and cognitive outcomes, highlighting a clear causal link between hypothalamic acetate levels and resilience to SF-induced ailments.
A key gene in this story is ACSS1, which is predominantly expressed in astrocytes, the star-shaped glial cells supporting neurons in multiple crucial ways. ACSS1 converts acetate to acetyl-CoA inside mitochondria, a critical step for channeling acetate into the TCA cycle for energy production and biosynthetic processes. Astrocyte-specific knockout of Acss1 (Acss1 cKO) prevented acetate utilization, leading to increased acetate accumulation in the hypothalamus. Interestingly, these mice were actually better off under chronic sleep fragmentation with improved glucose regulation and cognitive performance compared to wild-type SF mice, suggesting that the system’s protective strategy hinges on retaining enough acetate in the hypothalamus. The interplay between local acetate accumulation and its metabolic fates thus represents a delicate balance, with too little acetate correlating with metabolic harm, while channeling or sequestering acetate in certain contexts appears to enhance resilience.
An additional line of experiments demonstrated the importance of astrocytes in the paraventricular nucleus (PVN) of the hypothalamus. Using viral tracing and electrophysiological patch-clamp techniques, investigators found that astrocytes in the PVN can influence long-term potentiation (LTP) in the hippocampus via neural projections connecting the hypothalamus to regions like cornu ammonis 1 (CA1) and the dentate gyrus (DG). Modulating Acss1 expression in PVN astrocytes was able to alter these neuronal activities, thereby establishing a direct link between hypothalamic astrocyte metabolism and hippocampal function. In further tests, overexpressing Acss1 in PVN astrocytes prevented time-dependent accumulation of acetate in the hypothalamus. This condition, surprisingly, led to worsened glucose irregularities and cognitive deficits compared to mice without that overexpression during sleep fragmentation. Such findings illustrate the intricate balancing act that the brain undertakes in regulating acetate metabolism. While the presence of acetate is beneficial to a certain threshold, the route by which it is metabolized or sequestered in astrocytes crucially determines whether it will exert its protective effects.
Mechanistically, the beneficial actions of acetate on glucose metabolism appear to be mediated in large part by an upregulation of glycolytic and TCA cycle pathways, specifically through the activation of pyruvate carboxylase (PC). In vitro experiments on primary hypothalamic astrocytes validated this mechanism: when the cells were provided with isotopically labeled glucose in the presence of acetate, they showed a marked increase in glucose uptake and glycolytic capacity, signaled by heightened extracellular acidification rates. This effect was reversible by inhibiting GLUT1, one of the main glucose transporters in astrocytes. However, the protein expression of GLUT1, key enzymes like hexokinase 2 (HK2), pyruvate dehydrogenase (PDH), and others did not fluctuate significantly; rather, it was the enzymatic activity of PC, PDH, and HK that changed in response to acetate exposure, with PC being most prominently affected. By knocking down PC and PDH in astrocytes, it became apparent that acetate’s primary route for boosting glucose metabolism was through PC. Acetate thus helps reroute glucose fluxes toward critical metabolic endpoints that restore energy balance, reduce inflammation, and facilitate better cognitive function.
Beyond that, the relationship between acetate and astrocytic metabolism also extended to glutamate, an important neurotransmitter and energy substrate. Under normal circumstances, glutamate can be converted to α-ketoglutarate (α-KG) via glutamate dehydrogenase (GDH), feeding into the TCA cycle. Although acetate treatment also increased the contribution of glutamate to TCA intermediates, no substantial changes were seen in the overall levels of glutamate or glutamine, or in the expression of enzymes like GDH and glutamine synthetase (GS). This suggests that acetate’s effect on astrocytes is specialized and targeted: it increases the activity of enzymes that funnel nutrients into energy-producing pathways without globally altering all aspects of astrocyte metabolism.
In the pursuit of explaining exactly how acetate activates PC, researchers turned to advanced computational techniques known as mixed-solvent molecular dynamics (MixMD). By modeling the human PC enzyme at atomic resolution, they identified three significant binding sites (sites I, II, and III) that acetate tended to occupy, with site III being the most energetically favorable. Deletion experiments removing amino acid segments in the critical region 782–831 of PC revealed how this portion of the enzyme was necessary for acetate to exert its activating influence. This structural detail opens possible therapeutic targets, given that modulating this specific segment could theoretically enhance or inhibit the enzyme’s capacity to facilitate glucose metabolism in astrocytes.
The significance of PC for metabolic resilience was then reinforced in vivo with mice lacking Acss1 in astrocytes. When pyruvate carboxylase was further knocked down in the hypothalamus of these Acss1 cKO mice, the beneficial glucose uptake and improved metabolic markers were diminished, underscoring that the synergy between acetate accumulation and PC activation is essential in mitigating the impact of chronic sleep fragmentation. Interfering with either the supply or utilization of acetate—or indeed diminishing the PC route—removes this adaptive shield, with metabolic and cognitive performance consequently deteriorating.
Adding to the therapeutic relevance, the researchers tested whether acetate supplementation can help mice that were already metabolically compromised beyond the realm of sleep fragmentation. In models of high-fat diet-induced obesity and in db/db mice, which are genetically prone to type 2 diabetes, the administration of acetate still improved glucose homeostasis and insulin sensitivity. Such results imply that the protective effect of acetate is not limited solely to the consequences of interrupted sleep; rather, its beneficial role might extend to other metabolic disorders with overlapping physiological disruptions.
To connect these insights in mice to the human condition, a small-scale clinical observation study was performed. Individuals with obstructive sleep apnea (OSA) and type 2 diabetes were found to have elevated plasma acetate levels compared to healthy controls and OSA patients without T2D. Although additional research is necessary to fully clarify the relationship between these parameters in human populations, preliminary evidence aligns with the notion that acetate levels reflect important shifts in metabolic function during chronic sleep disruption. Genome-wide association studies (GWAS) offer further tantalizing clues. By analyzing data from large cohorts, investigators identified significant correlations between genetic variants in the Acss1 locus and traits such as fasting glucose, insulin, HOMA-IR, and T2D. These findings raise the possibility that genetic predispositions affecting acetate metabolism could influence the severity of sleep disorders and their attendant metabolic consequences. It also broadens the potential translational scope of interventions that target acetate or its metabolic pathways.
Taken together, all of these discoveries paint an arresting and scientifically exciting picture of how the brain and body react when sleep becomes disrupted for prolonged periods. Sleep fragmentation initiates a cascade of physiological events that culminates in insulin resistance, impaired glucose tolerance, and cognitive decline. Yet the body attempts to compensate by elevating acetate levels in the hypothalamus, thereby partially restoring metabolic flexibility and neuronal function. Indeed, blocking or reversing acetate’s beneficial presence leaves animals more vulnerable to these harmful outcomes, while boosting acetate or curtailing its use through Acss1 or PC manipulations can provide a protective advantage.
This phenomenon extends the common understanding that gut microbiota composition plays a foundational role in health, adding new layers to the concept of a gut-brain axis. In normal circumstances, SCFAs produced by gut bacteria are used in peripheral tissues or remain in the gut. Under chronic sleep fragmentation, damage to the intestinal epithelium apparently allows more acetate to traverse into the bloodstream and ultimately into the hypothalamus, where it accumulates and confers benefits. Although increased gut leakiness and dysbiosis are usually considered harmful, this phenomenon hints that the body may adaptively use the by-products of the microbiota to support neural integrity when faced with the stress of disturbed sleep cycles.
While these outcomes are far from an endorsement of chronic sleep disruption, they open a new perspective on the dynamic nature of metabolic control during adverse conditions. The beneficial influence of acetate on astrocyte function in the hypothalamus reveals an unanticipated resilience factor that might well be harnessed for therapeutic purposes. The capacity of acetate to modulate key enzymes in the glycolytic and TCA pathways, especially PC, underscores the intricacies of metabolic regulation. The protective effect of acetate is likely multifaceted, combining an anti-inflammatory response, improved insulin signaling, and enhanced energy production in crucial brain regions.
It is also critical to note that the interplay among ACSS1, astrocyte metabolic networks, and neuronal function is finely tuned, and interventions might need to be carefully calibrated. Indeed, merely increasing the activity of ACSS1 can prove counterproductive in certain contexts, as seen in experiments where overexpression of Acss1 in PVN astrocytes worsened SF-induced deficits. These findings highlight the role of astrocytes not just as passive supporters of neurons but as active mediators of metabolic resources, helping the brain adapt under conditions of distress.
The broader implications for human health are substantial. Sleep fragmentation is not only a characteristic of OSA, but it also occurs in shift workers, in many older adults experiencing age-related sleep changes, and in individuals with chronic conditions such as neurodegenerative diseases. If indeed acetate represents an adaptive mechanism to defend against insulin resistance and cognitive decline, therapeutic interventions might be designed to modulate acetate production, distribution, or utilization in targeted ways. Such an approach could complement existing treatments for sleep disorders, which typically focus on improving sleep quality and respiration (in the case of OSA), or on mitigating secondary consequences like daytime sleepiness and diminished alertness. Another potential application is in the area of metabolic syndromes, particularly since obesity and diabetes frequently coexist with sleep disruption in a complex interplay of mutual exacerbation.
Furthermore, the discovery that PC can be activated by acetate offers a novel strategy for restoring metabolic flexibility in astrocytes. Traditional interventions for metabolic disorders often revolve around controlling dietary intake, physical activity, and pharmacological manipulation of glucose or lipid pathways. By contrast, specifically targeting the acetate-PC interaction opens a new conceptual space in metabolic medicine. Future research might focus on small molecules that bind to the 782–831 region of PC, effectively mimicking the activation caused by acetate, or on carefully designed prebiotics and probiotics that encourage the growth of beneficial gut bacteria capable of producing metabolically favorable SCFAs under certain conditions.
Of course, critical questions remain. The net effect of chronic intestinal permeability on systemic health is generally negative, as it can lead to increased levels of circulating endotoxins (e.g., lipopolysaccharides) and inflammatory responses that overshadow any local metabolic advantage gleaned from enhanced acetate transport. Clarifying how these conflicting processes balance out will be crucial for devising safe therapeutic strategies. Another area in need of thorough investigation is the role of chronotype, circadian rhythm genetics, and how these overlap with sleep fragmentation. Understanding whether the protective role of acetate is circadian-phase dependent or whether certain circadian disruptions might negate its benefits could prove vital in translating these mouse model findings to humans with varying sleep-wake cycles.
The complexity of bridging the gap between lab-based findings and clinical practice also includes ensuring that interventions that elevate acetate or alter astrocyte function do not produce unintended consequences in other organs or systems. Since acetate is involved in multiple biochemical pathways, including cholesterol and fatty acid synthesis, an overabundance might have unpredictable metabolic ramifications over the long term. Hence, while these breakthroughs significantly advance our understanding of how the body responds to sleep fragmentation, they also underscore the need for rigorous, carefully controlled trials and a stepwise translational approach.
Despite these considerations, the overall message is both surprising and hopeful. Sleep fragmentation, long lamented for its role in driving metabolic dysfunction, may paradoxically spur a protective mechanism in the form of hypothalamic acetate accumulation. By elucidating how acetate is generated, transported, and metabolized—particularly in the astrocytes of the hypothalamus—this study reframes the narrative of sleep disruption from one of unmitigated pathology to a more nuanced interplay between damage and defense. It highlights the astounding adaptability of physiology: even under conditions of stress and disruption, the body deploys certain metabolites that help maintain balance and preserve function where possible.
This theme resonates with other areas of sleep research that reveal how different forms of sleep loss can trigger a variety of homeostatic or allostatic mechanisms. The new findings specifically implicate the gut-brain axis as a major player in orchestrating metabolic rescue strategies, with astrocytes at the center of the action. By forging a mechanistic link between changes in gut microbial communities, intestinal barrier function, SCFA levels in circulation, and hypothalamic metabolic signaling, the work extends beyond the conventional boundaries of neurology and endocrinology. It exemplifies the systems biology perspective that has gained traction in modern biomedical research, where health and disease states emerge from interactions among various organ systems, microbial flora, and environmental conditions such as sleep patterns and diet.
Moreover, the translational dimension of these findings is further strengthened by the genetic association analyses in humans. The negative genetic correlations identified between acetate and various blood glucose-related traits—fasting glucose, fasting insulin, HOMA-IR, and T2D—suggest that some individuals may possess variants in the Acss1 locus that predispose them to more robust protective acetate signaling during sleep fragmentation or other metabolic stressors. The interplay between these genetic factors, sleep quality, and broader lifestyle variables (like diet and exercise) is only beginning to be understood, underscoring a potent area for personalized medicine. In the future, it may be possible to screen for particular polymorphisms in Acss1 and related genes to identify individuals who would benefit most from an acetate-based therapeutic approach, especially if they are at risk of or already experiencing sleep fragmentation and metabolic instability.
Ultimately, the appeal of this research lies in its convergence of different scientific disciplines—sleep medicine, metabolism, microbiology, neuroscience, and molecular biology—to arrive at a cohesive picture of how the body copes with adversity. At a time when many people worldwide suffer from insufficient or poor-quality sleep, and when metabolic diseases reach epidemic proportions, illuminating even a single beneficial mechanism provides hope for novel treatments. The notion that gut-derived metabolites, often overshadowed by the more central hormones and neurotransmitters, can strongly impact brain function and protect against cognitive decline is an exciting frontier. It reemphasizes the value of maintaining a healthy gut microbiome and offers a glimpse into how targeted manipulation of SCFAs might help break the dangerous cycle of sleep disruption fueling metabolic dysfunction and vice versa.
Despite the positive perspective offered by these insights, the fundamental message remains that chronic sleep fragmentation is detrimental, and the best preventive measure is, of course, to prioritize good quality sleep. Strategies that enhance airflow during sleep (for those with obstructive sleep apnea), consistent bedtime routines, and interventions that mitigate circadian misalignments all remain paramount. However, for those who have difficulty implementing these ideal measures, or for whom metabolic conditions have already progressed, the prospect that acetate-driven pathways could help buffer against further damage is an important consideration for future medical research and development.
What began as a straightforward question—how does chronic sleep fragmentation harm the body?—has led to a nuanced answer that melds multiple areas of biomedical science. The discovery of acetate as a protective factor against SF-induced dysglycemia and cognitive impairment adds a critical dimension to our understanding of sleep regulation and metabolic resilience. By binding and activating pyruvate carboxylase, acetate alters glucose flux within astrocytes and offsets several of the detrimental outcomes of poor sleep, including hyperglycemia, inflammation, and memory deficits. This research not only identifies a novel protective mechanism but also sparks interest in translational opportunities, from dietary interventions that modulate gut-derived SCFAs to pharmacological agents that specifically target the acetate–ACSS1–PC axis in the hypothalamus. The vital interplay between the gut microbiome, intestinal permeability, and brain metabolism under conditions of disturbed sleep has been spotlighted, paving the way for innovative treatments that harness the natural defenses the body employs in dire circumstances. While many questions remain about the optimal levels of acetate, the precise timing of interventions, and the role of individual genetic differences, the overarching takeaway is one of cautious optimism: even in the face of chronic sleep disruption, the body retains hidden strengths that can be bolstered for better metabolic and cognitive outcomes.
Subject of Research: Sleep disruption, metabolism, and cognitive function in relation to acetate’s protective effects
Article Title: Acetate enables metabolic fitness and cognitive performance during sleep disruption
News Publication Date: January 17, 2025
Article Doi References: https://doi.org/10.1016/j.cmet.2024.07.019
Image Credits: Scienmag
Keywords: sleep disruption, acetate, glucose homeostasis, cognitive performance, pyruvate carboxylase, hypothalamus, astrocyte
What's Your Reaction?
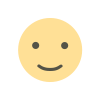
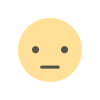
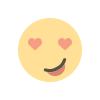
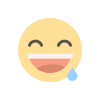
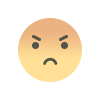
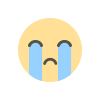
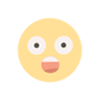