3D Hypersonic Simulation Unveils Novel Disturbances
At hypersonic speeds, the physics of fluid dynamics take a complex turn, influencing how gases interact with the surface of hypersonic vehicles. This interaction is defined by boundary layers, shock waves, and various unsteady flow characteristics that researchers are now beginning to demystify. At the forefront of this research is the Department of Aerospace Engineering […]

At hypersonic speeds, the physics of fluid dynamics take a complex turn, influencing how gases interact with the surface of hypersonic vehicles. This interaction is defined by boundary layers, shock waves, and various unsteady flow characteristics that researchers are now beginning to demystify. At the forefront of this research is the Department of Aerospace Engineering at The Grainger College of Engineering, University of Illinois Urbana-Champaign. Here, pioneering simulations are shedding light on previously unobserved flow patterns, all encapsulated within a remarkable 3D framework.
The researchers, led by Deborah Levin and her Ph.D. student Irmak Taylan Karpuzcu, have made significant strides in understanding the intricate flow behaviors around cone-shaped bodies at extreme speeds. High-fidelity 3D simulations require immense computational resources, which have traditionally restricted explorations in this domain. However, leveraging state-of-the-art machinery such as Frontera—the National Science Foundation-supported leadership-class supercomputer—has enabled Levin and Karpuzcu to push the boundaries of what’s computationally feasible, marking a remarkable advancement in aerospace engineering research.
The simulations reveal that transitioning flows are inherently three-dimensional and unsteady, a complexity not thoroughly explored until now. Many previous experiments conducted in the early 2000s lacked sufficient sensor coverage around cone-shaped models, thus failing to capture critical 3D dynamics. Karpuzcu highlights that while those early endeavors were valuable, they were limited by the technological capabilities of the time. With today’s advancements, the research team has gained access to a comprehensive dataset, revealing intricacies in the flow that were once obscured.
They specifically investigated the flow characteristics at a Mach number of 16 and observed unexpected disruptions in the flow field. This was contrary to the anticipated concentric flow patterns typically expected around a cone. The identification of these breaks in the flow was primarily concentrated near the cone’s tip, coinciding with heightened shock wave activity, where gas density increases significantly. Karpuzcu discusses the implications of increasing Mach numbers, stating that as this number rises, the shock wave escalates in proximity to the surface, encouraging instabilities that demand further scrutiny.
Despite the complexity of simulating high speeds, Karpuzcu mentioned that running the simulation at Mach 6 did not reveal the flow disruptions they noted at higher speeds. This pivotal finding indicates a distinct transition in flow behavior that is critical for the design of future hypersonic vehicles. By studying how flow properties vary with speed, the researchers hope to inform better design decisions that could ultimately enhance vehicle performance and safety.
Karpuzcu further explicates that the cone geometry mimics many configurations utilized in real hypersonic applications. When understanding the relationship between flow behavior and surface characteristics at hypersonic speeds, researchers can derive essential insights that feed into the engineering considerations for future vehicle designs. This ongoing research provides a foundation upon which aerospace engineers can build more efficient and effective hypersonic vehicles.
One of the most significant aspects of their research journey has been the efficient use of in-house software capable of harnessing parallel processing. Karpuzcu notes that this advancement has dramatically expedited their computational processes. The pre-existing experimental data under high-speed conditions offered a solid baseline, yet it was the full 3D simulations that unveiled unprecedented insights into the flow, including unexpected breaks.
The task of rationalizing the observed flow disruptions was not without its challenges. Karpuzcu reflects on the analytical phase of the project, discussing how the initial goal was to ascertain why such breaks were occurring. Their extensive literature review pointed them towards applying a linear stability analysis grounded in the triple-deck theory to correlate with their findings. This connection allowed them to develop a new computational code for simulating the flow problem comprehensively.
Utilizing direct simulation Monte Carlo (DSMC) techniques, the researchers achieved a robust simulation that accurately tracked individual air molecules through the flow field. This nuanced method stands in contrast to traditional computational fluid dynamics approaches, delivering a stochastic perspective wherein the behavior of gas molecules becomes a matter of probabilistic outcomes. Karpuzcu elaborates on the merits of DSMC, noting that this enables the tracking of billions of particles within the system, guaranteeing an accurate portrayal of particle interactions and collisions.
Karpuzcu expresses excitement about the potential that their findings hold not only for practical applications but also for advancing theoretical understanding in hypersonic flow dynamics. The interplay of technology and simulation has unlocked new doors in aerospace research, one that has previously been constrained by hardware limitations. As they continue to refine their methodologies and analyses, the knowledge generated promises to influence future engineering designs markedly.
A study recently published in the journal Physical Review Fluids delineates these findings, titled “Loss of axial symmetry in hypersonic flows over conical shapes.” Conducting research in this sphere is not just about identifying anomalies; it’s also about contributing to a deeper understanding of fluid mechanics that could transform the design and functionality of hypersonic vehicles. The collaborative efforts of established researchers and emerging scholars underscore the significance of advancing aerospace engineering through rigorous, cutting-edge research.
In conclusion, this groundbreaking exploration into hypersonic flow dynamics opens up new avenues for future innovations in aerospace technology. With each simulation, the complexities of air flow are unraveled just a little further, contributing invaluable insights that can no longer be overlooked in the realms of science and engineering. As researchers continue to push the boundaries of computational power and fluid dynamics understanding, the implications for future aerospace ventures are both exciting and promising.
Subject of Research: Hypersonic flows and their complexities
Article Title: Loss of axial symmetry in hypersonic flows over conical shapes
News Publication Date: 7-Mar-2025
Web References: Link to the study
References: N/A
Image Credits: The Grainger College of Engineering at the University of Illinois Urbana-Champaign
Keywords: Hypersonic flows, fluid dynamics, shock waves, Monte Carlo simulation, aerospace engineering, computational fluid dynamics, boundary layers, flight dynamics, 3D simulations, particle tracking, academic research, engineering design.
Tags: 3D hypersonic fluid dynamicsadvancements in 3D flow pattern analysisboundary layer interactions at hypersonic speedscomputational challenges in hypersonic researchcone-shaped body flow dynamicsDeborah Levin aerospace researchhigh-fidelity simulations for hypersonic vehiclesinnovative simulation techniques in engineeringNational Science Foundation supercomputing in aerospaceshock wave behavior in aerodynamicsUniversity of Illinois hypersonic studiesunsteady flow characteristics in aerospace engineering
What's Your Reaction?
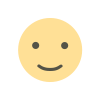
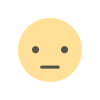
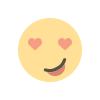
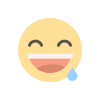
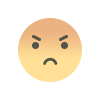
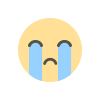
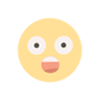