25.1% Efficient Inorganic-Organic Tandem Solar Cells
In the dynamic realm of photovoltaic research, perovskite solar cells have long been celebrated for their remarkable efficiency gains and cost-effective manufacturing potential. Yet, as the field ventures into advanced architectures like monolithic tandem devices, particularly those integrating wide-bandgap perovskites, researchers continuously confront formidable material and interface challenges. A recent breakthrough by Han, Fu, Ren, […]

In the dynamic realm of photovoltaic research, perovskite solar cells have long been celebrated for their remarkable efficiency gains and cost-effective manufacturing potential. Yet, as the field ventures into advanced architectures like monolithic tandem devices, particularly those integrating wide-bandgap perovskites, researchers continuously confront formidable material and interface challenges. A recent breakthrough by Han, Fu, Ren, and colleagues, detailed in Nature Energy (2025), has unveiled a novel bottom contact engineering strategy that significantly propels the performance and stability of inorganic perovskite/organic tandem solar cells, achieving a certified power conversion efficiency exceeding 25%.
Wide-bandgap perovskites, especially all-inorganic compositions such as cesium lead iodide bromide (CsPbI₂Br), are prized for their suitability as top-cell materials in tandem solar cells. Their larger bandgaps improve photovoltage and tandem efficiency, yet their practical implementation is vexed by issues including uncontrolled crystallization processes, abundant defect states, energetic misalignment at interfaces, and phase instability. These problems tend to originate predominantly at the critical interface where the perovskite layer contacts the underlying electron transport layer, frequently a tin oxide-based material.
Tin oxide (SnO₂) has emerged as a popular electron transport layer due to its optical transparency, high electron mobility, and thermal stability. However, when utilized in its conventional alkaline-based solution-processed form, SnO₂ often induces deleterious effects to the overlying perovskite film. The alkaline environment disrupts stoichiometry and crystallization dynamics, promoting defect formation and undesirable phase transformations. These interfacial imperfections exacerbate non-radiative recombination and voltage losses, ultimately dampening device performance and operational durability.
To overcome these hurdles, the authors crafted an innovative bottom interface modulation approach by synthesizing acidic magnesium-doped tin oxide quantum dots. This acidic nanomaterial sculpted a more benign and optimized contact surface conducive to the nucleation and growth of high-quality CsPbI₂Br thin films. Unlike traditional alkaline SnO₂, this magnesium-doped variant delicately balances the physical, chemical, structural, and energetic properties of the interface. This synergy results in profound passivation of trap states, significantly reducing carrier recombination pathways.
The magnesium doping not only adjusts the acidity of the quantum dot dispersion but concurrently fine-tunes the energy band alignment between the electron transport layer and the wide-bandgap perovskite. This precise energetic matching facilitates efficient electron extraction and minimizes energy losses at the interface. Furthermore, the controlled acidic environment promotes uniform perovskite film morphology with larger grain sizes and fewer pinholes, key factors that support enhanced charge transport and suppression of defect proliferation.
Delving deeper into the instability mechanisms previously encountered with alkaline-based tin oxide contacts, the study reveals that the basic nature of the conventional SnO₂ solution provokes deleterious chemical interactions at the interface. These reactions destabilize the CsPbI₂Br lattice and trigger phase transitions that deteriorate device longevity. By contrast, the acidic magnesium-doped SnO₂ quantum dots not only foster superior initial film quality but also fortify the structural integrity against environmental and operational stresses.
Performance metrics cement the remarkable improvements afforded by this bottom contact engineering innovation. The standalone wide-bandgap CsPbI₂Br perovskite solar cell achieves a striking power conversion efficiency of 19.2% alongside a high open-circuit voltage (V_oc) of 1.44 V, exemplifying substantial reduction in voltage losses. This milestone embodies a significant leap over prior records hampered by interfacial defects and instability.
Extending this advancement to the tandem format, the researchers seamlessly integrated the improved inorganic top cell with an optimized organic bottom cell, yielding a monolithic perovskite/organic tandem solar cell exhibiting an impressive efficiency of 25.9%, certified at 25.1%. This achievement ranks among the highest certified efficiencies reported for perovskite-based tandems and signals a promising future for these hybrid photovoltaic platforms.
Beyond sheer efficiency, the tandem device manifests enhanced long-term stability under diverse environmental conditions, spanning thermal, humid, and illumination stress tests. Such robustness is critical for transitioning laboratory innovations into real-world applications, where operational durability remains a primary concern.
The authors’ work exemplifies the transformative impact of interface chemistry and nanoscale material engineering on next-generation photovoltaic technologies. By challenging conventional electron transport layer paradigms and introducing a finely tuned acidic magnesium-doped SnO₂ quantum dot system, the research community gains a potent toolkit to tackle persistent instability and performance bottlenecks inherent to wide-bandgap perovskite devices.
This bottom contact modulation strategy not only signals a promising route to push power conversion efficiencies closer to their theoretical maxima but also invigorates tandem solar cell architectures with the stability and performance reliability necessary for commercialization. Given the ongoing quest for cost-effective, high-efficiency solar energy conversion, these findings resonate broadly across academia and industry, attracting attention from materials scientists, chemists, and photovoltaic engineers alike.
Moreover, the study provides a compelling mechanistic framework elucidating how subtle shifts in solution pH and quantum dot doping radically alter interfacial interactions, crystallization kinetics, and energetic alignments. Such insights will undoubtedly spur further exploration into tailored interface materials and nanoscale design strategies to address analogous challenges in other optoelectronic devices.
In summary, the research by Han et al. delivers an elegant and effective solution to one of the most pressing challenges in wide-bandgap perovskite solar cells. The use of acidic magnesium-doped SnO₂ quantum dots as a bottom contact modulator achieves a harmonious balance of chemical compatibility, structural optimization, and energy band alignment. This balance yields perovskite films with reduced defects, enhanced stability, and superior photovoltaic performance.
The resultant high-efficiency tandem device, combining the merits of inorganic perovskites with organic photovoltaics, underscores the versatile potential of hybrid architectures. As the solar industry strives for sustainable, efficient energy solutions, such scientific breakthroughs offer compelling pathways toward scalable, durable, and high-performing solar cells.
Looking ahead, the interface engineering paradigm illustrated here opens avenues for integrating other dopants and fine-tuning interface acidity/basicity to engineer bespoke electron transport layers tailored for diverse perovskite and tandem compositions. This flexible approach promises to accelerate the development of commercial-grade solar cells capable of meeting global energy demands with improved cost-effectiveness and reliability.
In essence, this study not only advances the state-of-the-art of perovskite and tandem photovoltaics but also enriches our fundamental understanding of interfacial chemistry in complex optoelectronic systems. The successful implementation of this bottom contact modulation strategy represents a major stride toward realizing the full potential of wide-bandgap perovskites in next-generation solar energy technologies.
Subject of Research: Interface engineering in wide-bandgap cesium lead halide perovskite solar cells and perovskite/organic tandem photovoltaics.
Article Title: Inorganic perovskite/organic tandem solar cells with 25.1% certified efficiency via bottom contact modulation.
Article References:
Han, Y., Fu, J., Ren, Z. et al. Inorganic perovskite/organic tandem solar cells with 25.1% certified efficiency via bottom contact modulation. Nat Energy (2025). https://doi.org/10.1038/s41560-025-01742-8
Image Credits: AI Generated
Tags: bottom contact engineering strategycesium lead iodide bromideelectron transport layer materialsinorganic-organic tandem solar cellsmonolithic tandem devicesperovskite solar cell efficiencyphotovoltaic research advancementspower conversion efficiency breakthroughssolar cell material challengesstability in tandem solar cellstin oxide in solar cellsWide Bandgap Perovskites
What's Your Reaction?
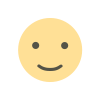
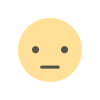
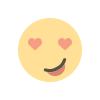
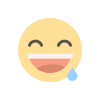
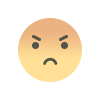
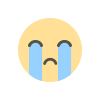
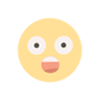